1. Introduction
Extreme ultraviolet (EUV) radiation covers the wavelength range of 5–40 nm and is of great importance to applications in EUV lithography[1–3], synchrotron radiation[4], solar astronomy[5], photon-level diagnostics[6], and free-electron lasers[7]. Freestanding filters are critical optical components with high EUV transmittance and efficient out-of-band suppression[8–11]. The absorption coefficient of the EUV photons of the filters must be as small as possible[12], and the filters are designed with hundred-nanometer thicknesses, leading to poor mechanical strength. Simultaneously, the energy of the absorbed EUV photons is sufficiently high enough to cause irradiation damage to the filters, thereby reducing filter lifetime and efficiency. Therefore, the addition of spacing and capping layers, such as Si, Nb, and [14–16], has been proposed to enhance the mechanical strength of the Zr-based filters and protect them from oxidation. In addition, the accelerated aging of the filters due to heat load, surface contamination, and exposure to intense photons requires better understanding.
Chkhalo et al.[13] studied the long-term heating by laser radiation onto the multilayer filters. They fabricated a filter coated with , and its transparency only decreased by 4.8%, while the uncoated one decreased by about 10%. To go further, Soer et al.[17] developed a grid Si filter with an EUV transmittance of 72% at normal incidence, which could stand a laser power density of for 8 h. Zuev et al.[18] reported on the fabrication and thermal stability of filters. The Be-based structures showed a high threshold for the absorbed power () from annealing. Long-term vacuum annealing of Mo/Be and Be ultrathin filters showed that they could withstand 24 h of vacuum heating without noticeable changes in EUV transmission. The stability of the layers during long-term vacuum heating was reported before by Gusev et al.[19], where freestanding absorption filters based on Mo/Si and were tested under conditions of long-term heating in a vacuum in the temperature range of 700°C–1000°C. The oxidation of the materials and sublimation of the Si at high temperatures were observed in the structure. Thus, a detailed analysis of the damage to the Zr-based filters under EUV exposure and in-depth research on the mechanisms of radiation damage and transmittance degradation are necessary to improve the performance of the filters.
In this Letter, we investigated the thermal stability of Zr filters under vacuum heating and the performance evolution of the filters under synchrotron radiation in the EUV range. The influence of the structures of the filters is studied. The transmittance of the filters in the EUV range after damage by radiation is measured. To discuss the mechanisms of the observed damage by radiation, a detailed component analysis of the filters was performed using X-ray reflectivity (XRR), X-ray photoelectron spectroscopy (XPS), and a scanning electron microscope (SEM).
Sign up for Chinese Optics Letters TOC Get the latest issue of Advanced Photonics delivered right to you!Sign up now
2. Experimental Details
Multilayer filters, including Zr/Si and were fabricated, and the structure of filters and images of samples are shown in Fig. 1. The period of the multilayer is 10. In each period, the thickness of the Zr is 30 nm, and the thickness of the interlayer and capping layer is 2 nm. The experimental thickness of the films was controlled by the sputtering rates and was determined by fitting the Bragg peaks in the XRR spectra. During the fabrication process of the filters, the films were initially deposited by direct current (DC) magnetron sputtering. Zr (99.95%), Si (99.999%), and (99.995%) targets were used for film deposition. The size of the targets was . The base pressure was less than , and the working gas was Ar at a constant pressure of 0.25 Pa. The coating substrate includes the crystal NaCl for the preparation of the filters and the Si wafer for the twins’ measurement XRR and XPS. After coating, the NaCl substrate was dissolved by liquid etching (in deionized water). In the final fabrication stage, the film was separated from the substrate and floated on the surface of the water. The floating film was then removed from the liquid and attached to the membrane.
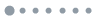
Figure 1.Schematic of multilayered filters (left). The difference between the two kinds of filters (right) is the material of the barrier and capping layers.
The application of filters in EUV lithography is limited by the risk of destruction from the degradation of their optical properties when heated to temperatures above 300°C by the radiation of the EUV[9]. Thus, the thermal stabilities of different structures were investigated. The twins’ samples that were deposited on the wafers were annealed in a high-temperature sintering furnace in a vacuum below 0.1 Pa and at temperatures up to 300°C. After annealing, the samples were characterized using XRR and XPS. The XRR was measured to analyze the thickness and interface evolution of the multilayer structures. It was performed on a PANalytical Empyrean X-ray diffractometer with Cu () at an energy of 8 keV. The elemental composition profiles and atomic percentages of the coatings were determined by XPS (Thermo Scientific K-Alpha) using a monochromatic Al (1486.6 eV) X-ray source and were performed on all samples before and after annealing. The estimated etching rates of the Zr, Si, and were approximately 0.07, 0.125, and 0.07 nm/s, respectively. The beam energy used for depth etching was 1 keV.
The measurement of the transparency of the filters and the EUV exposure was performed at the spectral radiation standard and metrology beamline (BL08B) of the National Synchrotron Radiation Laboratory (NSRL). A pure Zr filter with a thickness of approximately 300 nm was fabricated as the reference. The spot size of the light beam was . The EUV transmittance of the filters was measured in the 5–20 nm range with a step size of 0.03 nm in the normal-incident mode. The irradiation of the filters involved two steps. First, the radiation was produced by a 13.5 nm EUV pulse with an incident peak power of approximately 5 µW. The durations of long-term exposure were 2, 5, and 17 h. Second, the filters were further irradiated by high-energy diffraction light with an energy of 5 mW to simulate the surface damage of the filters when the gratings of the beamline were scanned across the zero order. The temperature distributions of the filters under irradiation were investigated using finite-element simulations[20,21]. The ex-situ surface morphology of the radiation-induced damage was observed using a BX53M Olympus optical microscope and a Carl Zeiss AURIGA CrossBeam SEM.
3. Results and Discussion
3.1. Thermal stability of the Zr filters
The thicknesses of the multilayer Zr/Si and films in the as-deposited and annealed states were determined by XRR. As shown in Fig. 2, the Bragg peaks of each sample have no significant shift when annealing at 300°C. The intensity of the peaks only slightly decreases after the first two hours, and then the peaks are flatter with continuous annealing. In the Zr/Si multilayers, the Bragg peaks almost disappeared after annealing owing to interfacial diffusion. The thicknesses of the individual layers are listed in Table 1. In the multilayer Zr/Si films, and . After annealing, the thickness of the Zr layer increased by 1.3 nm after the initial two hours before decreasing by 0.1 nm after 12 h, which confirms the mechanism of thermally induced diffusion between the Zr and Si layers[22]. The decrease in the thickness of Si also indicated that diffusion proceeded from the Si layers to the Zr layers. On the contrary, in the multilayer films, the intensity of each Bragg peak dropped and broadened, leading to the fitted thickness of the Zr layer decreasing from 30.0 to 29.2 nm at the end of the annealing process. In contrast, the thickness of the layer increased by approximately 0.9 nm. These results prove that thermal loading leads to a reverse diffusion direction from the Zr to the layers at the interface.

Table 1. Fitting Parameters of the Coated Multilayer Films from the XRR Curves (unit: nm)
Table 1. Fitting Parameters of the Coated Multilayer Films from the XRR Curves (unit: nm)
| Zr/Si | Zr/B4C |
---|
dZr | dSi | dZr | dB4C |
---|
as-deposited | 29.1 | 1.4 | 30.0 | 1.0 | 2 h-annealed | 30.4 | 1.2 | 29.9 | 1.4 | 12 h-annealed | 30.3 | 1.0 | 29.2 | 1.9 |
|
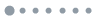
Figure 2.XRR results of (a) the Zr/Si and (b) the Zr/B4C multilayer films after annealing. The fitting results of the structures are listed in Table 1.
Depth-profile XPS measurements were performed to investigate the distribution of several elements near the film surface and characterize the protection provided by the cap structure. The samples were measured before and after being annealed at 300°C for 2 h. The etching ended below the surface, where the concentration of Zr 3d reached a stable plateau. The concentration profiles of the elements, including Zr 3d, O 1s, Si 2p, B 1s, and C 1s, are shown in Fig. 3 as functions of the etching time. Owing to the difference in the etching rates of the film layers, the operating times of the three samples were slightly different. As shown in Fig. 3(a), a noticeable oxidation of the Zr monolayer structure occurred during heating. The etching time corresponding to the same Zr and O concentrations increased from 60 to 280 s after annealing. The maximum atomic percentage of the Zr in the as-deposited Zr film was 81%. As shown in Fig. 3(b), in the multilayer Zr/Si films, the atomic percentage of the Zr in the bottom layer was less than 80% owing to the intermixing caused by the bombardment effect at the Si-on-Zr surface. Furthermore, the large concentration of ZrSi compounds at the Zr-on-Si interfaces is identical to that observed in earlier research[23]. After annealing, the oxygen concentration in the Zr layer increased by approximately 5%. A possible explanation is that the ZrSi is sufficiently stable at the interface below the surface, limiting the reaction between Zr and O to a deeper range. As shown in Fig. 3(c), in the multilayer samples, the peak of B 1s had a lower sensitivity and coincided with the peak position of Zr 3d, which could no longer be identified after the peak of Zr 3d increased. Therefore, the main elements beneath the first layer were Zr 3d, O 1s, and C 1s. After annealing, even with a stable period structure characterized by the XRR, the atomic percentage of O in the bottom layer increased significantly from 11% to 22%. These findings suggest that Si-capped multilayer filters are more stable under heating than -capped multilayer filters.
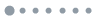
Figure 3.XPS depth profiles for O, Zr, Si, B, and C near the film surface. (a) Zr, (b) Zr/Si, and (c) Zr/B4C. The dotted lines illustrate the profiles of the as-deposited films, and the solid lines illustrate the profiles of the annealed films.
Figure 4 shows the regional XPS spectra of the Zr 3d peaks measured before and after annealing. The positions around the interfaces correspond to etching for 75 s (Zr), 480 s (Zr/Si), and 640 s () in depth-resolved scanning. At the surface of the as-deposited Zr films [Fig. 4(a)], the peaks at 178.4 and 180.7 eV can be identified as the Zr and Zr energy levels of the pure Zr metal. In addition, the peaks of the oxides (Suboxide I) and (Suboxide II) are also found with chemical shifts of and [24], respectively. After annealing, full zirconium oxide () with a chemical shift of 4.6 eV was observed. The absence of pure Zr peaks and the increasing intensity of the oxide peaks of , , and indicate severe oxidation of the Zr. In the as-deposited Zr/Si multilayer structure [Fig. 4(b)], the peaks with a chemical shift of 1.0 eV were identified as ZrSi at the interface. After annealing [Fig. 4(e)], no oxide peak was found at the interfaces. Therefore, the change in the O content in Fig. 3 can be assumed to be a change in the proportion of silicon oxides. The intensity of the Zr metal peak decreased and that of the ZrSi compound increased, implying that the thermal load led to intermixing between Si and Zr. However, as shown in Figs. 4(c) and 4(f), suboxide peaks at 181.5 and 184.0 eV were observed at the multilayer interfaces, indicating that the fails to prevent the oxidation of the Zr. Like the multilayer Zr/Si films, peaks of the (180.9 and 190.3 eV) compounds were observed at the interface of the multilayer films independent of annealing, and the intensity of the peaks increased after annealing.
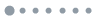
Figure 4.Deconvolution of the Zr 3d peak of each film on the interfaces corresponding to the etching time of 75 s (Zr), 480 s (Zr/Si), and 640 s (Zr/B4C). In particular, (a)–(c) as-deposited films and (d)–(f) after annealing at 300°C for 2 h.
3.2. Transmittance of filters in the EUV range
The transparencies of the Zr, Zr/Si, and filters are shown in Fig. 5. The Zr filters exhibit a higher peak transmission, approximately 28.7% at . In contrast, the multilayer filters show a slight decrease in transmittance owing to the significant absorption coefficients of the Si (L-absorption edge at 12.5 nm) and the (K-absorption edge at 6.63 nm). The L-absorption edge of the Si results in more significant transmission loss for the multilayer Zr/Si filters in the spectral range of 6.8–12.5 nm and a higher transmittance than the filters after 12.5 nm.
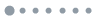
Figure 5.Transmittance curves of (a) Zr, (b) Zr/Si, and (c) Zr/B4C filters. The graphs show the comparison between the calculated and measured values of transmittance for different samples. The blue curves are fitted by IMD.
In the wavelength range of 7.5–20 nm, the measured transmission curves of the filters are different from the calculated transmission curves, which use parameters obtained from XRR in Sec. 3.1. Therefore, the calculation structures were modified in the IMD, including the primary layers of Zr, Si, and , and an individual layer of at the interfaces. Because C has been suggested to be an inevitable contaminant in synchrotron systems, the layer of C at the surfaces was also considered. The modified curves in blue color in Fig. 5 fit well with the measured transmission curves. After modification, a 22.78-nm-thick layer of was used on the surfaces of the Zr filters. In the multilayered filter structure, was simulated at the interface between Zr and the interlayers. The introduction of the Si and spacers restrained the oxidation of the Zr. The total thicknesses of the for the Zr/Si and filters were only 11.28 and 15.61 nm, respectively.
3.3. EUV radiation damage experiment
To study the aging of the filters induced by EUV radiation, a long-term (2–17 h) experiment was performed at the synchrotron radiation beamline at the NSRL, Hefei, China. Figure 6 shows the in situ measurement of the transmittance of the filters. Interestingly, the transmittance of all filters increased by approximately 1% after the first two hours of irradiation with monochromatic light at 13.5 nm (see Table 2). The observed increase in the transmittance of the filters after irradiation could be attributed to the desorption of the water and oxidation from the surface, although the vacuum of the experimental chamber in the synchrotron radiation facilities is as high as but is not able to isolate water. After some first hours of irradiation, the transient thermal effect caused a clean process of the water and oxidation on the filter surface, resulting in an increase of about 1% in the transmittance of the EUV light source. A decrease in the transmittance of the filters was recorded over the next three hours. The transmittance of the Zr filters was 1.3% lower than the initial value, whereas the multilayer filters remained higher than the original value. Following continuous long-term irradiation for 5–17 h, the transmittance of the filters decreased by approximately 2%. These results agree with the findings in previous studies on decreasing transparency after heating[13,25]. Compared to the pure Zr filter, the transmittance of the multilayer Zr/Si filters was relatively stable. Only a 0.1% decrease in transmittance at 13.5 nm after long-term radiation of the multilayer Zr/Si filters was observed, whereas the transmittance of the filters decreased by 3%. Although the multilayer filters exhibited a transmittance curve similar to that of the pure Zr filters, their transmittance decreased significantly during irradiation, indicating their lack of stability under irradiation.

Table 2. Measured Transmittance of Zr and Multilayered Filters at λ = 13.5 nm Before and After Irradiation by 13.5 nm EUV and High-Energy (HE) Particles
Table 2. Measured Transmittance of Zr and Multilayered Filters at λ = 13.5 nm Before and After Irradiation by 13.5 nm EUV and High-Energy (HE) Particles
Irradiation time (h) | Transmittance (%) |
---|
Zr | Zr/Si | Zr/B4C |
---|
0 | 28.5 | 26.8 | 23.0 | 2 | 29.8 | 28.0 | 24.3 | 5 | 27.2 | 27.4 | 23.5 | 17 | 26.8 | 26.7 | 20.9 | HE | 25.4 | 24.2 | 19.3 |
|
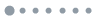
Figure 6.Filter transmittance curves under 13.5 nm EUV irradiation over 17 h. (a) Zr, (b) Zr/Si, and (c) Zr/B4C.
This study employed zero-order diffraction light to compare high-energy-source-induced damage to the filters. This light covered photon energies both lower and higher than that of the EUV, including X-ray, visible light, and various high-energy particles, and its intensity dramatically exceeded that of the monochromatic EUV. The filters were irradiated with zero-order diffracted light for one hour. As shown in Table 2 and Fig. 7, the transmittance of the multilayered Zr/Si filters decreased by 2% even with the capping layer, indicating that the filters underwent rapid oxidation after high-energy irradiation.
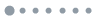
Figure 7.Filter transmittance after irradiation with 5 mW high-energy light.
3.4. Structure and component evolution induced by EUV radiation damage
After irradiation, including 17 h of EUV and 2 h of zero-order diffraction light, the noticeable ex situ damage morphologies of the irradiated filters were observed using optical microscopy and an SEM, as shown in Fig. 8. Without protection from the capping layer, the radiation damage to the Zr filters [Fig. 8(a)] was noticeable. An ablation crater with a lateral size of approximately 1.5 µm formed at the irradiation center. In contrast, only a different level of contamination deposition on the damaged surface compared to the undamaged area was observed in the multilayered filters [Figs. 8(b) and 8(c)]. From the SEM images, two types of regions can be distinguished: Region 1 contains the undamaged area of the Zr filters, where the crystallization of the Zr is visible, and Region 2 contains the damaged areas, where the surface is relatively opaque because of a continuous film of C contamination at the surface after exposure. The state of C is different in all three samples. It is a continuous film in the Zr filters [Fig. 8(a2)], discontinuous films in the multilayer Zr/Si filters [Fig. 7(b2)], and an islanded growth with an average size of 1 µm in the filters [Fig. 8(c2)]. The contamination in [Fig. 8(c)] was more markable than that in Zr/Si [Fig. 8(b)], proving that C contributed to the decrease in transmittance of the filter under long-term irradiation.
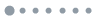
Figure 8.Left column: (a)–(c) optical microscope images of the damage produced by irradiation. Middle column: (a1)–(c1) SEM images of the undamaged areas of the filters. Right column: (a2)–(c2) SEM images of the damaged areas of the filters.
To explain the mechanisms of the observed damage, the finite-element numerical simulations of the thermal accumulation on the filters were performed. Filters with the structures discussed above were simplified to a cylindrical model with the bottom surface facing a vacuum environment for heat exchange and with thermally insulated sides. The filters were set at room temperature () at the beginning of the simulations. To simplify the temperature-rise modeling, the temperature dependence of the thermo-optical parameters was neglected. The thermal parameters of the relevant materials in the Zr-based filters are listed in Table 3, where , , and are the mass density, heat capacity at constant pressure, and thermal conductivity, respectively.

Table 3. Parameter Values Used in Finite-Element Numerical Simulations
Table 3. Parameter Values Used in Finite-Element Numerical Simulations
Material | Zr | Si | B4C |
---|
Density, ρ (g/cm3) | 6.49 | 2.33 | 2.52 | Specific heat capacity, Cp [J/(kg/K)] | 270 | 700 | 1020 | Heat transfer coefficient, κ [W/(m · K)] | 22.7 | 150.0 | 121.4 |
|
The spatial energy distribution of the beam is typically Gaussian, and the EUV power was set to 5 µW at a normal incidence. Subsequently, zero-order diffraction light irradiation was simulated with identical parameters, but with a power of 5 mW. The simulation results are listed in Table 4. The temperature of the thermalized filters increased from room temperature (293 K) to approximately 327 K under EUV irradiation and then to approximately 400 K at the end. The difference between the final temperatures of the three filters was smaller than 2 deg. Thermal diffusion is not sufficiently high enough to produce damage processes such as melting or mechanical fracture[26] but can accelerate the aging of materials (such as oxidation).

Table 4. Simulated Surface Temperatures (unit: K) of Zr-based Filters Irradiated by EUV and Zero-Order Diffraction Light
Table 4. Simulated Surface Temperatures (unit: K) of Zr-based Filters Irradiated by EUV and Zero-Order Diffraction Light
Sample | Zr | Zr/Si | Zr/B4C |
---|
Initial state | 293.1 | 293.1 | 293.1 | Exposed to EUV | 327.2 | 327.4 | 326.2 | Exposed to zero-order diffraction light | 402.3 | 403.1 | 400.1 |
|
The XPS survey spectra of the filter surfaces were given to identify the chemical bonding states of the elements, including C, O, Zr, and Si. Before the measurement, the freestanding filters were transferred to the Si wafers. Due to the poor flatness of the transferred samples, the depth-etching process and the element concentration were not considered. In Fig. 9, the peaks at 181.1 and 185.0 eV can easily be identified as the Zr 3d of the pure Zr metal and , respectively. Both filter surfaces showed C contamination, which was adjusted to 285 eV as the calibration energy for all spectra to compensate for the surface charge effect.
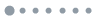
Figure 9.XPS survey spectra for all filters after irradiation. The binding energy ranges between 0 and 700 eV, where only the C 1s and O 1s peaks are observed in the multilayered filters.
The component evolution of the filters was further explained using the reported phase diagrams of the materials[27,28]. According to the Zr–B–O phase diagram and the XPS discussion in Sec. 3.1, as the percentage of Zr decreases, a transition from the ZrB to the phases is formed. Simultaneously, the increasing temperature of the filters will also add the proportion of , thereby producing more free C atoms. The Zr–Si–O phase diagram shows that the resulting Zr silicide is in a stable phase in this system, even when partial oxides are formed, owing to their lower formation energy.
4. Conclusion
The components and structural evolution of the Zr filters induced by annealing and synchrotron radiation were discussed in detail. Transmittance measurements of the filters are carried out at 5–20 nm wavelengths, and a 1% increase of the filter transmittance is observed after 2 h of irradiation by 13.5 nm EUV light. It could be related that some first hours of irradiation could induce a cleaning process of the water and oxygen on the filter surface, compared to the morphology caused by synchrotron radiation, long-term EUV, and zero-order diffraction light irradiation, which led to damage of the surface of the filters and decreased their EUV transmittance. Carbon was found in the irradiated filter surfaces, with the difference that it formed a continuous film in the Zr filters, a discontinuous film in the multilayer Zr/Si filters, and an islanded growth with an average size of 1 µm in the filters. The degradation mechanism of the Zr filters was further analyzed through annealing and finite-element simulations. The addition of Si improved the thermal stability of the Zr/Si filters, as reflected by the relatively stable state of the Zr 3d peaks at the interface of the filters after annealing. By comparing the phase diagrams, it was found that a sufficiently stable ZrSi compound formed at the interface below the surface, limiting the reaction between Zr and O in a deeper range and improving the stability of the multilayer filters. The reaction of the film with Zr formed and free C atoms, leading to a relatively disordered state.
The experimental results presented in this Letter will help to understand the degradation mechanism and mitigate the contamination of EUV filters and advance their promising applications in synchrotron radiation, astronomic observations, and EUV lithography.