Introduction
Since the first demonstration of laser diode (LD) on group Ⅲ-nitride materials some 20 years ago, technology has significantly matured with the availability of high quality GaN and, recently, AlN substrates. This led to the demonstrations of high-performance laser diodes ranging from infrared to visible and down to the UV spectral regime claiming their applications in high-density data storage, spectroscopy, sterilization, chemical detection, communications, and manufacturing industry1, 2. However, the development towards deep UV (< 300 nm) lasers remains a great challenge mainly due to the lack of high quality single crystalline native AlN substrate. On the other hand, AlGaN devices grown on foreign substrates such as sapphire and silicon carbide suffer higher threading dislocation densities up to 1010 cm-2 and reduce the internal quantum efficiency. In addition, strain in AlGaN epitaxial layers due to lattice mismatch forms cracks when layers exceed critical thickness3-5. This results in an increase in non-radiative recombination centers, higher threshold and lower slope efficiency.
Optically pumped lasers based on AlGaN/AlN heterostructures at deep UV have recently been demonstrated by some groups using the MOCVD growth technique. Most of the reports are based on 3 to 10 periods of MQWs with threshold power densities ranging from 1200 kW/cm2 down to 6 kW/cm2. In 2004, a 3-pair 5 nm/10 nm Al0.66Ga0.34N/Al0.76Ga0.24N MQWs LD structure was demonstrated by Takano et al at 241.5 nm with threshold of 1200 kW/cm2 grown on 4H-SiC substrate6. Wunderer in 2011 reported a 3-pair AlGaN MQWs laser structure with threshold of 126 kW/cm2 at 267 nm7. In 2014, Guo et al reported a 10-pair 1.6 nm/4 nm Al0.5Ga0.5N/ Al0.7Ga0.3N MQW edge emitting laser at 265 nm with threshold of 150 kW/cm28. The per QW threshold is calculated to be 15 kW/cm2, but the full width half maximum (FWHM) is 1.8 nm. Most recently in 2018, R. Kirste et al. reported a 3-period 2.5 nm Al0.55Ga0.45N/AlN MQW laser at 267 nm with low threshold power density of 6 kW/cm2 by reducing unintentional point defects in the active region through high supersaturation growth conditions for waveguide layer. However, the FWHM is still approximately 1 nm 9. The threshold power density was decreased by increasing the Al composition in barriers thus increasing the barrier height to confine the carriers within the wells. These trends show a reduction in threshold power density by reducing the point defects, increasing the barrier height and optimizing the number of QWs and their thickness. However, the narrowest reported lasing linewidth is around 0.8 nm4, 10. Similar to the threshold power density, the linewidth can also be further narrowed down with better growth quality of AlN epitaxial layers, thus increasing the laser characteristics.
In this work, we report a 21-period 2 nm Al0.53Ga0.47N/6 nm Al0.7Ga0.3N MQWs optically pumped laser on bulk AlN substrate by low pressure organometallic vapor phase epitaxy (LP-OMVPE) in a high-temperature reactor5, 11. The results show a dominant deep UV laser peak at 280.3 nm, with lasing threshold of 320 kW/cm2 and lasing FWHM of 0.08 nm at room temperature. Such a narrow spectral linewidth and low lasing threshold demonstrate high quality 21 QWs heterostructure growth.
Laser cavity design and fabrication
Shown in Fig. 1 is the complete laser structure grown on an AlN substrate by LP-OMVPE in a high-temperature reactor. Following an initial 400 nm AlN homoepitaxial layer on the AlN substrate, a Si-doped 600 nm n-Al0.74Ga0.26N contact/cladding layer was grown prior to the 21-period 2 nm Al0.53Ga0.47N/6 nm Al0.7Ga0.3N MQWs active region. XRD was carried out to verify the material growth quality. Figure 2 shows the high-resolution XRD reciprocal space map. The measurement was carried out along (002) the direction with high-resolution triple axis scans. As shown in the RSM for a 21 AlGaN QW grown on AlN substrate, high quality pseudomorphic growth was achieved with very close agreement with simulation for a completely pseudomorphic structure. Figure 3 shows the simulation of mode profile in the heterostructure with 3, 15, and 21-period MQWs. This helps us to understand the confinement factor in the QWs and in turn the gain threshold value. We observed increments in the confinement factor as the number of QWs were increased. The heterostructure was designed to obtain a large modal confinement factor in the QW region. For the same heterostructure design, the optical mode confinement factor is stronger for 21 QWs with 18.51% for TE mode and 14.35% for TM mode respectively compared to 3 and 15-period QWs. Figure 5(b) shows the peak PL emission intensities of heterostructure designs with 3, 15 and 21 MQWs at different pumping power densities. It is observed that for a given pump power density, the peak PL emission increased linearly with the number of QWs. Therefore, 21-period MQW structure is used for the laser device fabrication.
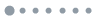
Figure 1.The deep UV laser heterostructure grown on AlN substrate with MQWs directly exposed to the air.
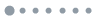
Figure 2.Pseudomorphic growth: High-resolution XRD reciprocal space map.
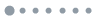
Figure 3.Simulations of TE and TM mode profile in the heterostructure with 3, 15 and 21-period MQWs.
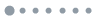
Figure 4.Schematic experimental setup to measure the edge emission from the cleaved facets.
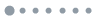
Figure 5.AlGaN QW growth on bulk AlN substrates.
(a) Photoluminescence spectra for different sets of QW designs with different numbers of QWs and different Al compositions shown in Table 1; and (b) Photoluminescence intensity for different sets of QWs.
The surface photoluminescence (PL) was measured to identify the QWs emission and gain region. A circular 193 nm laser beam of 800 μm diameter was incident on the sample and emission was collected at 45° with respect to the incident beam. Figure 4 shows the surface photoluminescence experimental set up. We tested different sets of QW designs to compare the peak intensity and gain region. Table 1 shows the heterostructure information of each sample including the number of QWs, Al composition in QWs and barriers. The measured PL spectra from each sample are shown in Fig. 5(a). The emission intensity increased with the number of QWs as shown in Fig. 5(b). We considered sample S5 with emission peak centered at 273 nm to process our laser device as the emission from S5 sample is stronger than all the other samples at similar testing conditions. It is approximately 1.77 and 2.25 times stronger than S4 and S3 samples respectively. A subsequent chemical mechanical polishing (CMP) process was performed to thin down the substrate to around 70 μm.

Table 1. Structure information for different sets of QW designs with different numbers of QWs and different Al compositions.
Table 1. Structure information for different sets of QW designs with different numbers of QWs and different Al compositions.
Sample | # of QWs | QWs composition AlxGa1-xN | QBs composition AlxGa1-xN | Peak wavelength (nm) | Sample name | S1 | 3 | 0.75 | 1 | 234 | HT3761 | S2 | 15 | 0.75 | 1 | 234 | HT5198 | S3 | 21 | 0.72 | 1 | 234 | HT5294 | S4 | 21 | 0.58 | 1 | 251 | HT5439 | S5 | 21 | 0.53 | 0.7 | 273 | HT5630 |
|
Results and discussion
The sample was then cleaved on one side to measure the net modal gain and loss using the variable-stripe length method. The stripe length is varied from 0.1 mm to 1 mm and emission from the facet is collected at various pump power densities. The onset of super-linearity is observed as the stripe length is increased from 0.5 mm, as shown in Fig. 6. The net modal gain is calculated by fitting the peak emission intensities vs stripe excitation length plot by using Matlab to the equation (1),
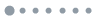
Figure 6.Peak emission intensities at different stripe excitation lengths for different pumping power densities. The onset of super-linearity after 0.5 mm excitation stripe length.
where IASE is the amplified spontaneous emission intensity at different stripe lengths land wavelengths λ, A is the constant related to the spontaneous emission intensity, and G is the net optical gain as a function of wavelength8. Figure 7(a) shows the gain spectra at different pump power densities. The net modal gain linearly increased with pump power up to 60 cm-1 and saturated from 500 kW/cm2, as shown in Fig. 7(b).
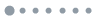
Figure 7.Optical gain measurement.
(a) Measured gain spectra at different pump power densities, and (b) peak net modal gain at different pump power densities.
Later, the other side was also cleaved to form two parallel facets, with a cavity length of 3.5 mm. The QWs were directly exposed to the air on the top side with Si-doped cladding on the bottom. The laser structure was optically pumped using a coherent 193 nm ArF deep UV excimer laser source with a 7 ns pulse width and 200 Hz repetition rate. Cylindrical lenses were inserted in the pumping beam path to control the pump beam shape and beam uniformity. All tests were carried out at room temperature. The emission was collected from one of the cleaved edge facets into a fiber cable and coupled into an iHR550 monochrometer of 2400 groves/mm grating with a spectral resolution of 0.01 nm. Figure 8(a) shows the measured spectral outputs at various pumping power densities with a pump beam width of 120 μm over the entire laser cavity length of 3.5 mm. Although the gain guided region is significantly broad, a dominant single mode lasing emission peak at 280 nm appeared above the threshold power density of 320 kW/cm2. Figure 8(b) shows the light-light (L-L) characteristics on primary y-axis and FWHM variation with pumping power density in secondary y-axis. The linewidth was observed to be 0.08 nm, much narrower than any other DUV lasers reported so far (typ. 0.8 nm)4, 10. An elliptical shaped far-field pattern from the MQWs region was observed on the UV sensor card above the threshold at pumping power density of 350 kW/cm2. The increase in the number of QWs has raised the threshold power density, but the threshold power density per QW is 15.2 kW/cm2 which is still low for AlGaN MQWs structure with AlGaN barriers. These results with the low per QW threshold power density and narrow linewidth suggest excellent QW heterostructure cavity and low optical loss in the processed laser structure.
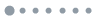
Figure 8.Optically pumped laser emission measurement.
(a) Edge emission spectra measured from the cleaved facet at different pump power levels. (b) Measured L-L curve and FWHM at different pumping power densities.
Polarization properties were also measured, as shown in Fig. 9. Below threshold, we observed TE dominated emission with a TE/TM ratio of 2. On the other hand, above threshold, the emission is 100% TE polarized without any TM modes. These results are also in agreement with earlier reported work indicating dominant TE mode above 240 nm12.
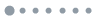
Figure 9.Polarization measurement (a) below threshold at 230 kW/cm2 and (b) above threshold at 350 kW/cm2.
Conclusion
We successfully demonstrated an optically pumped 280 nm deep UV edge emitting laser under pulsed operation at room temperature with a threshold of 320 kW/cm2 and lasing linewidth of 0.08 nm. The threshold per QW is only 15 kW/cm2, suggesting high-quality crystal growth and processed laser cavity.
Acknowledgements
The work was supported by Defense Advanced Research Projects Agency (DARPA) under grant # HR0011-15-2-0002. The program managers are Dr. Daniel Green and Dr. Young-Kai Chen.
Author contributions
Akhil Raj Kumar Kalapala, Dong Liu, Sang June Cho and Jeongpil Park contributed equally to this work.
All authors commented on the manuscript.
Competing interests
The authors declare no competing financial interests.