1. Introduction
Organic-inorganic hybrid halide perovskites have been widely applied in solar cells[1,2], light-emitting diodes (LEDs)[3,4], and photodetectors[5–7]. Compared to 3D perovskite, low-dimensional perovskite is more prone to anisotropic luminescence and photo-response due to the reduction of at least one dimension[8], which is essential in numerous display and photodetection applications[9–11]. For example, Li et al. observed a polarization-dependent emission evolution and an enhanced self-trapped exciton emission with an oblique incident excitation from the cross-plane in BA2PbI4 2D perovskites[12]. Jurow et al. found strongly anisotropic light emission from symmetric CsPbBr3 perovskite nanocrystals on a range of surfaces, and the degree of anisotropy of the emitted radiation can be controlled by modulating the interaction between the substrate and the nanocrystal surface to control particle spacing[13]. Li et al. reported an optimized photodetector composed of 2D crystals, demonstrating a high responsivity and detectivity. Interestingly, the photocurrent of this photodetector varies as the angle of the incident polarized light increases[14].
On the other hand, for perovskite LEDs, the majority of the radiative recombination generated within the emissive layer is trapped inside the thin-film stack through the dissipating pathways of the waveguide, the surface plasmon, and the substrate modes. It is pivotal to boost the light outcoupling efficiency () to enhance their external quantum efficiency (EQE). Among all the methods to increase the , using oriented emitters with the transition dipole moments parallel to the substrate is an efficient approach[15]. There are many such examples in perovskite LEDs[16–18]. Kumar et al. reported that a direct self-assembly approach to form the anisotropic nanocrystal superlattices can increase the ratio of the horizontal dipole up to 0.75 with the increase of the EQE to 24.96%[16]. Ye et al. showed the direct generation of linearly polarized electroluminescence (EL) with a high degree of polarization of 74.4% through the use of strongly confined nanoplatelets[17]. Cui et al. achieved efficient EL from oriented perovskite nanoplatelets. The ratio of horizontal transition dipole moments of the nanoplatelet film is , which leads to a higher light-outcoupling efficiency () than that of the isotropic emitters ()[18].
Compared with polycrystalline and owing to the higher stability, lower defect density, and higher carrier mobility, perovskite single crystals have drawn increasing attention for optoelectronic applications instead of polycrystals[19–21]. Moreover, single crystals are also an ideal platform for studying the intrinsic physical properties of perovskite materials due to the long-range ordered structure and minimal defects[22–24]. single crystals have stronger structural anisotropy. Liu et al. manipulated the photoluminescence (PL) emission in using a diamond anvil cell[25]. Owing to the strong anisotropic deformation of the single crystal, an ultrabroad tunable emission had been observed, starting from 2.38 eV (0 GPa) and reaching full quenching at 1.84 eV (). In addition, when the PEAI was used as the organic ligand, the 2D perovskite may exhibit stronger sensitivity to polarized light[26–28]. Hence, the investigation and modulation of anisotropic luminescence and the photocurrent of low-dimensional perovskite single crystal are pivotal for perovskite optoelectronic devices. In this work, the anisotropic luminescence and photocurrent were systematically investigated in single crystals. An external electric field was also used to control the exciton behavior to modulate the luminescence. This work will deepen our understanding of the exciton physics of perovskite and promote the development of highly efficient LEDs and polarized photodetectors.
Sign up for Chinese Optics Letters TOC Get the latest issue of Advanced Photonics delivered right to you!Sign up now
2. Results and Discussion
Two-dimensional perovskite single crystals were synthesized by the anti-solvent vapor-assisted space-confined growth method[29], which confined the perovskite solution between two substrates and used dichloromethane as the anti-solvent. The crystal structure of is shown in Fig. 1(a), and the X-ray diffraction (XRD) pattern [Fig. 1(b)] reveals the equally spaced planes with the crystal axis aligning perpendicularly to the crystal planes and the substrate[30]. The XRD pattern shows that the crystal grows along the (001) direction, indicating that the crystal phase is very pure[31]. Compared with the lattice parameters of the crystal at room temperature[32], the crystal system remains unchanged at a low temperature (Fig. S1 in the Supporting Information), but the lattice parameters and unit cell volume slightly decrease. Using classical mechanical exfoliation methods, we obtained crystal nanosheets with flat surfaces and homogeneous optical quality on the substrate and covered it with boron nitride (BN) as an insulator [Fig. 1(c)]. The thickness of the crystal is , and the thickness of the BN is (Fig. S2 in the Supporting Information). The minimal surface fluctuation and low defect level diminish the inhomogeneous broadening of optical spectra, allowing for the observation of the exciton fine structure at 78 K [Fig. 1(d)].
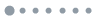
Figure 1.(a) Crystal structure diagram of PEA2PbI4. (b) X-ray diffraction spectrum of (PEA)2PbI4. (c) Optical microscope image (top) and fluorescence image (down) of a representative exfoliated sample. (Green dotted area: (PEA)2PbI4; yellow dotted area: BN.) (d) Spectral comparison at 78 K and room temperature.
The relationship of the excitation power-dependent PL intensity can help us discriminate between the types of excitons corresponding to the recombination processes. The process can be described by , where is the excitation power, and is the emission intensity. The four emission peaks (P1, P2, P3, and P4) all rise linearly with the increase of laser power with k values of 0.94, 0.94, 0.94, and 0.95, respectively [Figs. 2(a) and Fig. S3 in the Supporting Information]. This implies that all the peaks should be related to neutral excitons. By comparing the PL characteristics of our samples and the previous studies[30,33,34], the high-energy peak P1, P2, and P3 are typically ascribed to free excitons, while that of P4 should be ascribed to defect-bound excitons. To explore the anisotropic PL of the PEA2PbI4 crystal, we built a polarization-resolved measurement [Fig. 2(b)]. In this device, a 405 nm half-waveplate was placed at the excitation light port to control the polarization of the excitation light (from 0° to 90°). In an attempt to probe the polarization-dependent phenomena, we added a half-waveplate before emission collection in the system. By rotating the half-waveplate (400–700 nm) before the polarizer, the polarized emission was checked as a function of the polarization angle. We defined the polarization state with the most intensive emission as 0°.
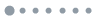
Figure 2.(a) Intensity of the four emission peaks varies with the excitation power, and linear fitting is adopted. (b) Experimental setup for polarization-resolved measurements. (c) Polarized PL spectra taken at 78 K at selected detection angles of β = 0° and 90° by fixing the excitation polarization to α = 0°. Inset: the relationship between the PL intensity of the P1 peak and the detection angle β fitted with the sine function. (d) Schematic diagram of the device with the loading of an electric field (E).
PL spectrum almost stayed the same while varying the incident laser-direction in the crystal plane (Fig. S4 in the Supporting Information). On the contrary, by fixing the excitation angle (), the PL spectrum changes dramatically with different detection angles [Fig. 2(c)]. The PL emission intensity is the strongest when being detected in parallel to the polarization axis of the polarizer (). It becomes minimal when the detection direction is perpendicular to the polarizer (). The trend of P1, P2, and P3 photoluminescence intensity as a function of the excitation angle is presented in the inset of Figs. 2(c) and Fig. S5 in the Supporting Information, verifying the linear polarization with the fitting of the sine function. At the same time, we also set up a control experiment to confirm that the half-waveplate before the emission collection system can further improve the anisotropic luminescence of the crystal. The spectrum of the whole system without the half-waveplate is almost the same as the spectrum with the half-waveplate only at the excitation light port, but when the half-waveplate is placed before the emission collection system, the polarization of the sample luminescence is further improved (Fig. S6 in the Supporting Information).
The variation of the polarization spectrum with the detection angle depends on the orientation of the dipole[35]. Next, we investigated the effect of the applied electric field on the polarization spectrum and the related orientation of dipoles. We fabricated the device with a capacitive structure based on the previous sample [Fig. 2(d)], using and BN as the insulating layer, using heavily n-doping Si as the bottom electrode, and using Au as the top electrode, and we utilized the source meter to provide applied voltage. There is no change in the spectra for 10 min, indicating the high stability of the single crystal and the stable temperature in the device, which can ensure the accuracy of the test data (Fig. S7 in the Supporting Information).
Since the half-waveplate placed at the excitation light port has no influence on the emission spectra, we fixed the excitation polarization to . In comparison with , when the detection angle was rotated to , the overall photoluminescence intensity was higher, but the voltage-dependent luminous intensity also decreased more quickly [Figs. 3(b), 3(e), and Fig. S8 in the Supporting Information]. P1, P2, and P3 peaks are all very narrow with no defect bunching characteristic and with very small energy separations of 3 meV for P1 and P2 and 6 meV for P2 and P3, indicating their origin as the exciton fine-structure splitting[30]. This is consistent with the results in Fig. 2(a). It is reported that the excitons at the single specific layer of two-dimensional organic-inorganic perovskite are influenced by the static electric force of the other layers in a thick perovskite single-crystal thin film[30,36,37]. This may influence the orientation of the in-plane excitons of the perovskites, leading to different voltage-dependent emission behavior changes in different peaks[38].
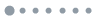
Figure 3.(a),(d) Voltage-dependent PL spectra. (b),(e) The changes in the PL intensity of the four peaks, and the changes in the ratios of P1, P2, and P3 at 78 K at selected detection angles of (a)–(c) β = 90° and (d)–(f) β = 0° at a fixed angle of excitation light (α = 0°).
In the following analysis, we will focus on the effect of the electric field on the dipoles with different orientations, namely, P1/P2, P1/P3, and P2/P3. The energy difference between P1 and P2 is small, but the energy difference with P3 is large [Figs. 3(a) and 3(d)], implying that P1 and P2 may have similar exciton orientation and/or behavior, while P3 may originate from the emission of excitons at different layers of lead iodine octahedra caused by the thicker sample with a different orientation[30,39]. Thus, the ratio of P1/P2 remains almost the same [Figs. 3(c) and 3(f)].
The electric field can induce the dissociation of excitons with the decrease of emission intensity. The overall tendency shows that the intensity of P3 decreases faster than those of P1 and P2 [Figs. 3(c) and 3(f)], which agrees well with the discussion in Figs. 3(a) and 3(d), i.e., that P3 may come from excitons at different layers from those of P1 and P2, and the orientation of the exciton for P3 may have a more out-of-plane component than that of P1 and P2.
In addition to, the anisotropic exciton behavior, free charge carriers can also show anisotropic characteristics in single crystals. As shown in Fig. 4(a), we made a simple horizontal detector. By changing the polarization angle α of the excitation laser from 90° to 0°, the photocurrent in the crystal will increase a lot, indicating the anisotropic charge carriers characteristics [Fig. 4(b)]. The photocurrent increases more quickly as the excitation angle () rotates to 0° [Figs. 4(c) and Fig. S9 in the Supporting Information]. At a higher irradiation intensity of , a significant photocurrent was generated in the crystal. Moreover, the photocurrent generated at is much larger than that generated at and 45° [Figs. 4(b) and Fig. S10 in the Supporting Information]. At a voltage of 30 V, when the angle () at the excitation light changes from 90° to 0°, the photocurrent increases by about 3 times [Fig. 4(c)]. At a voltage of , when α changes from 90° to 0°, the photocurrent increases up to 11 times [Fig. 4(d)], which is larger than [14]. All these experimental results are reproducible and reversible (Fig. S11 in the Supporting Information). According to the report, the polarization-sensitive photodetection was dominant by the anisotropic absorption owing to the different electronic states caused by the anisotropic crystal structure[14]. This result indicates the existence of photocurrent anisotropy in the crystal, which may be used in the polarization photodetector and to enhance the photocurrent in perovskite solar cells.
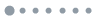
Figure 4.(a) Schematic illustration of the detector. (b) Photocurrents under different voltages at different excitation polarizations. Photocurrents at different excitation polarizations at (c) 30 V and (d) −30 V.
3. Conclusion
In this work, polarization-resolved equipment with different excitations and detection polarizations of light are set up. Anisotropy of luminescence and photocurrent and the electric field-dependent luminescence behavior were found in the single crystal. Three narrow peaks and one broad peak with a strong anisotropy and an energy separation of 3, 6, and 10 meV were observed at 78 K, originating from neutral excitons. The three narrow peaks are related to exciton fine structure splitting. P1 and P2 excitons have similar behavior, while that of P3 shows more out-of-plane components, which were dissociated the most by the electric field. Interestingly, we found that there exists an anisotropy of photocurrent in a horizontal detector: crystal/Au. When the polarization angle () of the excitation light is adjusted from 90° to 0°, the photocurrent in the crystal can be greatly increased, indicating the anisotropic characteristic of the free photogenerated charge carriers in the single crystal. This work is important for the fundamental investigation of exciton physics in perovskites, as well as the development of highly efficient LEDs and polarization photodetectors.
4. Experimental Section
Preparation of single crystals: The perovskite precursor solution (1 M) was prepared by dissolving PEAI (99.5%) and (99.5%) at a molar ratio of PEAI : : 1 in anhydrous dimethyl sulfoxide (98.0%, Sigma-Aldrich) and stirred overnight at room temperature. The precursor solution was filtered through 0.22 µm filters before use. Two quartz substrates were cleaned with deionized water, isopropanol, and acetone in an ultrasonic bath for 10 min and then treated by plasma for 10 min. One substrate is placed at the bottom of a big Teflon cap vial, and 10 µL of the solution was deposited on the substrate and was immediately capped by the second quartz substrate. Finally, 1 mL of DCM in a small vial was placed at the top of the substrates. After serval hours, rectangular-shaped crystals appeared in between the two substrates. The PEAI and were purchased from Advanced Election Technology Co., Ltd.
Device fabrication[40,41]: The sandwich-structure device used the mechanical peel-off technique to obtain the crystal, the BN, and the graphene with nanoscale thickness sequentially. Then, under the microscope, The crystal was transferred to a substrate containing a gold electrode, covered with a thin BN, and then lastly, graphene was overlaid on the crystal and the Au electrode. For the detector, the mechanically exfoliated crystal was placed on a substrate with a pair of parallel Au electrodes, then encapsulated with a BN sheet. The distance between the two Au electrodes is about 30 µm.
Optical measurements: PL spectra were obtained from a micro-confocal Raman spectrometer (RENISHAW, Invia) with a dry objective (). The PL signals were stimulated by a 405 nm continuous-wave (CW) laser. Low-temperature PL measurements were performed by a temperature-controlled probe station (INSTEC, HCP421V-PM) with liquid nitrogen.
Electric measurements and structure characterization: The voltage applied in the device was generated from a SourceMeter (KEITHLEY 4200). The thicknesses of the crystal and the BN were characterized by an atomic force microscope (Bruker, Dimension ICON). The XRD pattern was obtained by a thin-film X-ray diffractometer (Bruker, XRD-7000) and single-crystal X-ray diffractometer (Bruker, APEX II).