1. Introduction
Cavity-less lasing actions of nitrogen, oxygen, and argon gas in ambient air pumped by intense ultrafast lasers were reported 10 years ago and have attracted growing attention due to their potential applications in optical remote sensing and the rich physics involved[1-3]. Due to the abundance of in atmosphere and the fact that the required driving laser wavelength falls into the widely available near-infrared (NIR) and mid-infrared (MIR) range, the study on the lasing effect of neutral and ionic nitrogen molecules has been exploited by many groups[3–6]. In the first report on the lasing action of nitrogen ions, a femtosecond MIR pulse in the range of 1.1 to 2.6 µm was employed. A series of coherent narrow band emissions at 391.4, 427.8, and 357.8 nm has been observed[3], which correspond to the optical transitions between the second excitation state (denoted as ) of nitrogen ions and its ground state (denoted as ), where and denote the vibrational quantum number. Later on, several groups reported that optical amplification occurs at these wavelengths in case of pumping with 800 nm femtosecond laser pulses[7-9]. In the past 10 years, many efforts have been devoted to the study on the nature of this radiation and the underlying mechanisms, as well as the applications of this lasing emission[10,11]. In case of the 800 nm femtosecond pulses, the radiation has been identified to be superfluorescence, based on its characteristic temporal profile and its dependence on gas pressure[12-15]. In particular, the 391.4 nm emission was found to lag behind the 800 nm pump pulse, with a pressure-dependent build-up time of several picoseconds[16]. In contrast, recently it has been revealed that in the case of MIR pumping, the 391.4 nm emission presents a duration of 2–3 ps, and there is no temporal delay between the pump laser and the radiation[6]. Based on its temporal features, it has been attributed to free induction decay (FID), a coherent emission process where the macroscopic coherence between the and states was established by the MIR pulse through a 3- or 5-photon resonance.
To optimize and enhance the lasing emission, several techniques have been employed in recent studies, such as pre-alignment of the molecules[17], polarization screwed light fields[18], and vector pump beams[19]. In 2023 the optical orbit angular momentum (OAM) was introduced to the study of nitrogen air lasing, and vortex nitrogen ion lasing has been demonstrated[19-21]. Specifically, with 800 nm pump lasers carrying OAM of , it has been found that the picosecond delayed 391.4 nm superfluorescence exhibits an identical OAM, highlighting the nature of coherent excitation of this emission process[19]. In the meantime, it has also been demonstrated that an injected seeding pulse with OAM into the nitrogen gas plasma created by a Gaussian 800 nm pulse can be amplified into an OAM beam[21]. Up to now, it is not clear whether the optical OAM plays a role in the FID process of nitrogen ions pumped with MIR pulses, especially in the highly nonlinear regime of light–matter interaction.
In this work, we pumped nitrogen ions with MIR pulses at 1850 nm with a topological charge of . Free induction decay at 391. 4 nm was observed, with its intensity less than that produced with conventional Gaussian beams. We examined the topological charge of the FID emission and found it equals 5, which can be explained by the FID being resonantly excited by a 5-photon process with the aid of the Stark effect. To the best of our knowledge, this constitutes the first observation of FID emission carrying OAM. This study opens the interesting perspective of multiple-photon resonant interaction of atoms and molecules with intense light field carrying OAM and provides a method to generate ultraviolet (UV) and extreme ultraviolet (XUV) pulses with high-order OAM.
Sign up for Chinese Optics Letters TOC Get the latest issue of Advanced Photonics delivered right to you!Sign up now
2. Experimental Setup
In the experiment, we employed an optical parametric amplifier (OPA, HE-TOPAS, Light Conversion Ltd.), which is pumped by a commercial femtosecond laser (Coherent Legend DUO). The femtosecond laser provides 35 fs, 800 nm pulses with a maximal pulse energy of 12 mJ at 1 kHz repetition rate. The whole output pulses were employed to pump the OPA system with a maximal conversion efficiency about 25% at signal pulse wavelength around 1300 nm. In our experiments, we used the idler pulses from 1700 nm to 1900 nm, with a pulse energy around 380 µJ and pulse duration about 60 fs. To impose a topological charge to the MIR pump pulse, we inserted a quarter-wave plate, a vortex half-wave plate, and a second quarter-wave plate, as shown in Fig. 1. This combination has been widely used for the generation of vortex beams[22]. We focused the vortex MIR pump pulses into a gas cell with a convex lens of . In the gas cell, a bright plasma string can be observed by naked eyes when the pump pulse energy goes beyond . The output emission from the nitrogen gas plasma was filtered by several filters to eliminate the fundamental pulse. The radiation of the nitrogen ions in the UV range was collimated and captured by a charge-coupled device (CCD) for observation of the spatial profile. To characterize the spectrum of the radiation, the emission was collected by a convex lens into a fiber tip connected to a spectrometer (Avantes, model AvaSpec-ULS3648-USB2). To analyze the topological charge of the MIR pump laser and the generated 391.4 nm signal pulse, we used a cylindrical lens with and recorded the beam profile at the focal plane with the CCD camera, which has been widely used for measurement of the topological charge of vortex beams[19,21,23].
3. Results and Discussion
In Fig. 2, we first present the spatial profile of a conventional Gaussian pump laser at 1850 nm and the spectrum of the corresponding emission from the nitrogen gas plasma. In this experiment, the optical elements for the vortex generation were removed from the beam path. The 1850 nm pump laser shows a nearly Gaussian beam profile, as presented in Fig. 2(a). In Fig. 2(b), the two peaks located at 391.4 and 386.5 nm correspond to the P and R branches of the transition from the state to the state of the nitrogen ions. This radiation was attributed to free induction decay between the and states, which is resonantly excited by the MIR pulse through a 5-photon process from to levels[6].
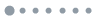
Figure 1.Experimental setup. QP1 and QP2 refer to quarter-wave plates for the 1850 nm pulses. L1 and L2 are convex lenses. CL is the cylindrical lens. The half-wave q-plate converts the incident circularly polarized beam into a circularly polarized vortex beam.
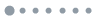
Figure 2.(a) Spatial profile of the Gaussian femtosecond MIR pulses with wavelength λ = 1850 nm. (b) Free induction decay signal of nitrogen ions pumped by the Gaussian MIR pulses. The gas pressure was 1 bar in (b).
We then examined the emission spectrum as a function of the pump laser wavelength with the Gaussian beam pump, with the results shown in Fig. 3. The pump pulse energy was fixed at 380 µJ in this experiment. It is seen that the 391.4 nm signal persists for the pump laser wavelength from 1700 nm to 1900 nm, with its intensity dependent on the pump laser wavelength. For pump laser wavelengths below 1780 nm, significant emission peaking at 357.8 nm was also observed, which corresponds to transition. We found that the optimal pump wavelength for the 391.4 nm signal was around 1850 nm. The deviation of the optimal pump wavelength from that of the field-free 5-photon resonance case () has been attributed by the alternating current (AC) Stark shift of the intense pump laser imposed on the nitrogen ions[5,6]. We noticed that the optimal pump laser wavelength in the current study presents a redshift with respect to the recent report where 1800 nm was found to produce the most intense FID signal[6]. This redshift can be related to the less significant AC Stark shift compared to the previous report[6]. Another feature is the absence of the Fano line shape in the current experiments, which was observed in the previous report[6,24]. It should be noted that the appearance of the Fano-type spectrum necessitates a subtle balance between two quantum channels, which requires an optimization of the pump laser intensity and gas pressure.
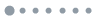
Figure 3.The emission spectrum as a function of the wavelength of the pump laser. The pulse energy of the pump laser was fixed at 380 µJ. The gas pressure was 1 bar.
We then turned to the study of the nitrogen ions emission pumped with the OAM beam. In Fig. 4(a), the profile of the 1850 nm beam with a topological charge of is presented, which shows a characteristic donut shape. To confirm its topological charge, we focused the 1850 nm beam with a cylindrical lens and recorded its profile at the focal plane. The typical two-lobe structure with one central stripe of minimal intensity in Figs. 4(b) and 4(c) confirms that a femtosecond MIR pulse with a vortex of was generated.
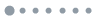
Figure 4.(a) Spatial profile of the vortex femtosecond MIR pulses with topological charge l = 1. The wavelength was 1850 nm. (b), (c) Profile of the vortex MIR pulses obtained with the CCD camera at the focal plane of the cylindrical lens f = 400 mm. In (b) and (c), the cylindrical lens was rotated to different angles θ. The stripes with minimum laser intensity in the center in (b) and (c) reflect that the MIR pulses possess a topological charge of l = 1.
In Figs. 5(a) and 5(b), we present the spatial profile and spectrum of the FID emission. A characteristic donut shape of the 391.4 nm emission suggests that it may possess a vortex phase structure. In Fig. 5(b), one noticed that the emission intensity is smaller than that in Fig. 2(b) by 1 order of magnitude. We speculated that this decreased emission intensity can be due to the reduction of pump laser intensity inside the gas plasma with respect to the Gaussian pump laser[25-27]. As is known, the laser intensity for a focused OAM beam with is less than that of a conventional Gaussian beam[25]. We measured the topological charge of the 391.4 nm emission with the cylindrical lens method, and the results are presented in Figs. 5(c) and 5(d) for different rotation angles of the cylindrical lens. Six bright lobes separated by five stripes are shown clearly in both figures, indicating that the emission has a topological charge of . We also numerically simulated the intensity profile of a vortex beam with at the focal plane of a cylindrical lens, and the results are presented in Figs. 5(e) and 5(f). The simulation parameters are taken to be close to those in the experiments. We considered that a collimated vortex beam with diameter of 3 mm was focused by a cylindrical lens of . The good agreement between Figs. 5(c), 5(d) and 5(e), 5(f) confirms that the 391.4 nm FID emission has a topological charge of 5.
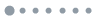
Figure 5.(a) Spatial profile of the FID signal at 391.4 nm from nitrogen ions. (b) Spectrum of the FID emission. (c), (d) Experimental results of the profile of the 391.4 nm emission taken by the CCD on the focal plane of the cylindrical lens f = 400 mm. The cylindrical lens was rotated by 45° from (c) to (d). (e), (f) Numerically simulated profile of a beam with topological charge of l = 5 at the focal plane of a cylindrical lens, which should be compared with the experimental results in (c) and (d).
How should we understand that the topological charge of the pump pulses was transferred to the 391.4 nm emission? Conservation of the topological charge in the case of optical harmonic generation is now well established and understood[28,29]. In this parametric process, the frequency of the emitted photons satisfies the relationship, , where is the number of the fundamental photons involved. The atoms or molecules do not absorb any optical angular momentum in this process, and the angular momentum is conserved for the photons. In the current case of FID emission, it has been reported previously that this 391.4 nm emission presents a 2–3 ps duration[6], much longer than the relatively shorter duration of the pump pulse around 60 fs. For the FID emission of the nitrogen ions pumped with MIR pulses, the quantum coherence between the B and X states is established by the fundamental light field through a multiple-photon process. After the passage of the femtosecond pump pulse, the macroscopic B-X coherence reaches its maximum and releases electromagnetic radiation immediately at the frequency determined by the field-free energy difference between the B and X states. This is an entirely coherent process, and the radiated electromagnetic wave is expected to inherit the phase information of the pump laser. In the case with pump laser at , due to the AC Stark shift of the intense pump laser with the intensity on the order of , the energy separation between the B and X states is increased by [6]. Here is the frequency of the pump laser. The difference of the 1850 nm photon energy (0.670 eV) with respect to the field-free 5-photon resonance wavelength 1957 nm (0.636 eV) matches well the Stark shift induced energy change by . Therefore, a 5-photon resonant excitation is responsible for the formation of the B-X coherence, and thus the FID emission possesses 5 times the topological charge of the driving MIR laser field.
We noticed that the optical FID emission in the UV range has also been observed in at 337 nm[6,30]. Moreover, free induction decay has been widely observed in the XUV range in Ar and metal plasma vapor[31-33]. Therefore, the method of optical excitation with pulses carrying OAM can provide a simple and direct technique to generate UV and XUV pulses with higher order OAM, which has been exploited for improved soft-X-ray microscopy[34], enhanced dichroism[35], generation of Skyrmionics defects[36], etc. In these spectral ranges, optical elements such as spiral phase plates (SPPs) and vortex half-wave plates are not commonly available.
4. Conclusion
In summary, we employed femtosecond MIR pulses carrying OAM () to pump nitrogen gas and investigated the optical FID at 391.4 nm emitted by the nitrogen ions. It was found that the 391.4 nm FID emission carries a topological charge of due to the 5-photon resonance. This proves that, in the FID process, the topological charge of the photons is conserved, although the FID emission presents a pulse duration of several picoseconds, in contrast with the duration of the pump laser. In addition, we found that the FID signal pumped by the vortex pump pulses was much weaker than that produced by the Gaussian beam, which can be attributed to the reduced laser intensity due to the presence of the vortex. A redshift of the optimal pump laser wavelength compared to the Gaussian beam can also be explained by the smaller AC Stark shift produced by reduced pump laser intensity. In view that the FID emission has been produced widely in the UV and XUV regime from atoms and metal plasma vapors, pumping with OAM pulses provides a simple and direct method to generate OAM pulses in the UV and XUV range.