1. Introduction
Attosecond sources based on high-order harmonic generation (HHG) in the extreme ultraviolet (XUV) and soft X-ray range have opened a route to measure and manipulate ultrafast electron dynamics at their intrinsic attosecond temporal scale and nanometer spatial scale[1,2]. HHG originates from the non-perturbative nonlinear laser–matter interaction[3]. Usually, it consists of a comb of odd-order harmonics of the driving laser, which corresponds to a train of attosecond pulses separated by the half-optical cycle of the driving laser in the time domain[4,5]. Even-order harmonics can also be generated when the inversion symmetry of the system is broken[6]. The generation of isolated attosecond pulse (IAP) was first demonstrated in 2001 using a Ti:sapphire driving laser[7]. A variety of gating techniques for IAP generation such as amplitude gating[8,9], polarization gating[10,11], ionization gating[12,13], double optical gating[14-18], and non-collinear gating[19,20] were developed[21,22]. Recently, owing to the advances in mid-infrared (IR) lasers, IAPs down to around 50 attoseconds were observed[23,24].
IAPs, as well as the attosecond pulse train (APT) directly from the HHG, have been successfully applied in time-resolved studies of electron dynamics, such as photoionization time delay[25,26], molecular dissociation[27,28], charge migration[29], and petahertz charge carrier motion[30,31]. Such light–matter interaction often involves multiple correlated photoelectrons and/or ions. These particles need to be recorded in coincidence in order to fully resolve the complex ultrafast process[32]. In coincidence measurements, the events produced by a single laser shot must be limited to at most one per shot. Ideally, a lower event rate is more desirable since the rate of false coincidence that contaminates the acquisition grows quadratically with the event rate[33]. The time-resolved techniques based on photoemission such as photoemission electron microscopy (PEEM) and angle-resolved photoemission spectroscopy (ARPES) also benefit from the low event rate to avoid the space charge effect[34]. Typical attosecond sources with a repetition rate of 1 kHz or less severely limit their application in this type of study because of the practical difficulty in the excessively long data acquisition time[35,36]. On the other hand, high-repetition-rate attosecond sources driven by fiber lasers or produced in enhanced-cavity have also been demonstrated[37,38]. Despite the high average power due to the high repetition rate, they are unfeasible for experiments that are demanding of single pulse energy. In this Letter, we report IAP generation driven by a carrier-envelope-phase (CEP) stabilized few-cycle near-IR (NIR) laser with output millijoule (mJ) level pulse energy at 10 kHz repetition rate at the attosecond laser station in the Synergetic Extreme Condition User Facility (SECUF)[39]. It is suitable for attosecond time-resolved coincidence measurements in atomic and molecular systems.
2. Methods
In this work, the driving laser for HHG was a Ti:sapphire chirped pulse amplification (CPA) system (Amplitude Technologies ARCO-W) seeded with 5 fs, CEP stabilized oscillator (Laser Quantum Venteon CEP5). It is a typical chirped pulse amplifier based on a grating stretcher and compressor, which delivers 1 mJ, 20 fs laser pulses with central wavelength of 800 nm at 10 kHz repetition rate. It was then coupled to a 300 µm hollow-core fiber filled with Ne to broaden the spectrum. The broadened spectrum spanned from 550 nm to 1000 nm with a transmission efficiency of 60%. The pulse was then compressed with chirped mirror pairs. The final output parameter of the laser was 0.5 mJ centered at 770 nm. The pulse duration was 5.2 fs measured by a D-scan device (Sphere Ultrafast Photonics SA)[40], as shown in Fig. 1. The Fourier transform limit supported by the spectrum was 4.8 fs. A small fraction of the broadband pulse after the hollow-core fiber was sent to an f−2f interferometer for slow loop CEP stabilization. The CEP jitter averaged in every 10 shots was about 0.4 rad. The CEP can be tuned by varying the insertion of the wedge mounted on a stage driven by a piezo actuator (Newport, Picomotor).
Sign up for Chinese Optics Letters TOC Get the latest issue of Advanced Photonics delivered right to you!Sign up now
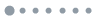
Figure 1.D-scan measurements of the driving laser pulse. (a) Measured and (b) retrieved D-scan trace for the compressed broadband laser pulse. (c) Measured spectral intensity (blue) and retrieved spectral phase (red) of the laser pulse. (d) Retrieved temporal profile of the 5.2 fs laser pulse (blue) and the Fourier transform-limited pulse supported by the spectrum (dashed red).
The schematic of the experimental setup is given in Fig. 2. The 5.2 fs, 0.5 mJ laser pulse was focused by an concave silver mirror to a gas cell made from a copper tube. The 1 mm gas cell was filled with Ne gas. The backing pressure was chosen to be 40 kPa to maximize the HHG output. The peak intensity of the focused laser was estimated to be , which corresponds to about 100 eV cut-off energy for HHG. A metallic foil was used to block the central part of the driving laser after the HHG. After the filter, the remaining HHG and the outer ring of the driving laser were sent to a double mirror for attosecond pulse isolation and characterization. The mirror was divided into two segments. The inner segment was a 3 mm concave mirror coated with multilayer Mo/Si. The reflectivity curve of the mirror is given in Fig. 3, and the central photon energy of the main peak was about 88 eV. The inner segment served as the bandwidth filter in the amplitude gating technique to extract the IAP from the HHG. The XUV continuum around 90 eV that supported an IAP was selected and focused by the inner segment. The outer segment was an annular concave mirror with the same focal length as the inner segment. The XUV and the outer ring of the NIR driving laser were focused by the two segments, respectively, to the gas jet in front of the time-of-flight (TOF) electron spectrometer (Stefan Kaesdorf ETF 11). The energy resolution of the TOF spectrometer was . The data acquisition was accomplished by time-to-digital converter in combination with constant fraction discriminator (RoentDek Handels GmbH). A toroidal mirror mounted on a motorized translation stage can be switched into the beam path to reflect the HHG at a grazing incidence angle for further application. The effective focal length of the toroidal mirror is 600 mm. It is designed to image the focal spot of the HHG region to a second interaction region, where a time-resolved pump-probe experiment in non-collinear geometry can potentially be performed. The HHG spectrum can be diagnosed by a flat field XUV spectrometer placed after the second focus.
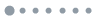
Figure 2.Experimental setup for generation and characterization of attosecond pulses. FM, focusing mirror; MA, motorized aperture; TM, toroidal mirror; DM, double mirror; TOF, time-of-flight spectrometer.
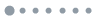
Figure 3.Reflectivity of the Mo/Si multilayer coated two-segment mirror utilized as the spectral filter in the amplitude gating of the HHG as a function of photon energy.
3. Results and Discussion
The temporal information of the IAP was characterized with the frequency-resolved optical gating for complete reconstruction of as bursts (FROG-CRAB) technique[41,42]. A 200-nm-thick Zr filter filtered out the low energy photons below 60 eV and helped for the compression of the atto-chirp. Due to the low damage threshold of Zr, the repetition rate of the driving laser was reduced to 2.5 kHz for long-term operation. While Zr offered the best pulse compression in our experimental parameters, a Ti filter can also support IAP generation when long-term and high-repetition-rate operation is required[43]. The collinear geometry of the XUV and NIR beams passively ensured the stabilization of the temporal overlap. The relative position, namely, the time delay of the reflected beams, could be tuned by a piezo stage on which the inner mirror was mounted with nanometer precision. The spatially and temporally overlapped XUV and IR beams were focused on the Ne gas jet with backing pressure of 100 kPa. The photoelectrons produced by the XUV beam and modulated by the NIR beam were detected by a TOF electron spectrometer. The intensity of the NIR field can be tuned by the motorized aperture before the filter. The estimated NIR intensity at the focus to perform the streaking measurement was . The photoelectron spectra were recorded as a function of the XUV-IR time delay. The step size was 167 attoseconds. The acquisition time for each step was 20 s. The recorded count rate of photoelectrons per laser shot was around 0.5. The continuum spectrum in the range of 70 to 100 eV that in principle supports a Fourier transform-limited 77-attosecond isolated pulse can be generated by tuning the CEP of the driving laser. A trailing edge at low photon energy, instead of the dip in the reflectivity of the Mo/Si mirror around 77 eV in Fig. 3, was observed. It is due to the higher transmission of the Zr filter towards higher photon energy and the limited energy resolution of the TOF spectrometer.
The measured streaking trace is shown in Fig. 4(a). An iterative loop based on least squared generalized projection algorithm (LSGPA)[44] was performed for the reconstruction of the amplitude and phase of the IAP. The retrieved spectrogram and temporal profile are given in Figs. 4(b) and 4(c). The full width at half-maximum (FWHM) pulse duration of the pulse was 86 attoseconds. The retrieved driving laser vector potential that was compared with a reference curve fitting to a 5.2 fs field is shown in Fig. 4(d). The retrieval is consistent with the D-scan measurement. While a prism-based CPA stretcher and compressor can lead to better CEP stability[45,46], we demonstrated IAP generation can also be realized by a grating-based CPA laser using amplitude gating that to a large extent relies on the CEP.
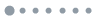
Figure 4.Retrieval of IAP and NIR pulses. (a) Measured and (b) retrieved FROG-CRAB trace of the IAP in the streaking field. (c) Reconstructed temporal profile of the IAP with an FWHM pulse duration of 86 attoseconds. (d) Reconstructed vector potential (blue) of the driving laser pulse and the 5.2 fs reference curve (red) according to the D-scan measurement.
4. Conclusion
IAPs were generated by applying an amplitude gating technique on the HHG driven by a 5.2 fs, CEP stabilized laser and characterized by the FROG-CRAB technique. The shortest pulse duration of the IAP retrieved from the attosecond streaking spectroscopy was 86 attoseconds at central photon energy of 88 eV using Zr filter. The attosecond source was developed for attosecond time-resolved photoelectron spectroscopy with the ability to perform coincidence measurements at a repetition rate of 10 kHz. Moreover, the development of the non-collinear arm of the system will enable the combination with 3D momentum measurement techniques, which is capable of studying the valence electron ionization, dissociation, and collision dynamics in atoms and molecules.