The exploration of high Q-factor resonances in the terahertz frequencies8 delves into their unique fingerprint features facilitating the enhanced molecular sensing, imaging, nonlinear photonics and strong cooperative light-matter coupling. The discovery9 of photonic BICs within the engineered structures further amplifies the potential of these high-Q resonances towards boosting the light-matter interactions in the vibrational and rotational-rich THz spectrum. Further, BIC in synergy with metasurfaces allows the ability to localize light, imparts intriguing topological properties and enhances the robustness, efficiency, and versatility of resonant photonic devices, thereby providing a strong foundation for next generation photonic solutions in the technologically significant THz domain. The notion of BIC represents a theoretical pinnacle, aiming to attain the utmost Q-factor and nonradiative mode within an ideal, non-lossy, symmetry-protected structure. In practical scenarios, one can strategically manipulate the symmetry of resonators, leading to the excitation of a leaky qBIC resonance with a high Q-factor. Notably, this resonance exhibits an inverse quadratic relationship between the Q factors and structural asymmetry6. There are challenges rooted in the THz frequencies related to material losses, dispersion characteristics, and fabrication imperfections, impeding the achievement of high-Q qBIC resonances. Specifically, the intrinsic and fundamental challenge of nonradiative/resistive losses in materials is a significant barrier, limiting the experimental realization of high Q-factors at THz frequencies. On the other hand, by carefully manipulating the radiative properties of the system, one can work towards minimizing losses and optimizing the conditions for sustaining high-Q qBIC resonances. This engineering effort involves tailoring the lattice/structural geometry10, composition11, and symmetry of the resonant structures to control the radiative behavior, ensuring that a significant portion of the energy is confined and prevented from being lost to the surrounding environment. Traditional strategies for accessing high-Q qBICs involved breaking symmetry at the individual resonator level in a uniform lattice6. However, in this work7 the researchers are now pushing the boundaries further, proposing a novel approach to manipulate radiative losses of the quasi-BIC resonances at the lattice level (in a hybrid/superlattice geometry) rather than at the resonator level, see Fig. 1(a-c). The effectiveness of this different strategy through selectively preserving the C2 symmetry of resonators in the supercell within a hybrid BIC lattice proves to be instrumental in reducing radiation density, thereby enhancing overall Q-factors of qBIC resonances at THz frequencies.
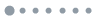
Figure 1.Hybrid lattices supporting quasi-BICs: (a) The schematic showing the cancellation of far-radiation field for the symmetry protected BIC structures. (b, c) The Fabricated samples showing the superlattice geometry of the Universal (U)-qBIC and Hybrid (H)-qBIC metastructures. (d) The universal inverse quadratic behaviour of the Q- factor of BIC system with respect to its assymetry paramater. (e) Experimetal THz spectrum showing the improved Q-factor numbers for the H-qBIC resonance compared to the U- qBIC resonance.
In photonics, the quest for high-quality (high Q) resonances driven by the physics of bound states in the continuum (BIC)1, 2 has motivated researchers to explore innovative avenues for realizing groundbreaking applications in lasing3, sensing4 and nonlinear photonics5. A conventional strategy to harness the properties of BICs involves breaking the symmetry of resonators in a uniform lattice, allowing uncoupled modes to interact with free space that opens a leaky channel in the form of so-called (quasi) qBIC6 modes. It is imperative that to unlock the full potential of photonic BICs, the approach should involve not only a fundamental understanding of their underlying physics but also innovative engineering strategies to tailor their properties for various applications. In a recent work7, a research team lead by Prof. Longqing Cong has introduced a more generalized scheme that leverages on selectively breaking the symmetry of only a fraction of resonators in a metasurface supercell to access qBIC resonance showing high Q-factors at terahertz frequencies. With a significant reduction in scattering losses and improved design robustness, this approach could open up new possibilities through innovative design strategies of the symmetry-protected BIC systems, potentially accelerating the development of practical applications in photonics and optoelectronics.
The team performed numerical simulations and experimental validations on an array of double split ring resonator (DSRR) structures, revealing the versatile engineering of symmetry-protected BICs. A key aspect of this design involved band folding analysis in the Brillouin Zone (BZ), providing visual insights into the evolution of modes and quality factors between uniform and hybrid lattices. In the hybrid lattice configuration, the team observed a selective retention of the high-Q portion of the band from the uniform lattice, while strategically discarding the low-Q portion during the band folding process. This resulted in a significant boost in the Q-factor, surpassing values more than 14.6 times higher than those observed in a uniform lattice in simulations and later verified by the experiments.
Another notable outcome of this work is the emergence of multiple Fano-type resonances at the Г point, accompanied by the band folding. The underlying physics driving this phenomena and improving Q factors of hybrid BIC supercells can be visualized in the reciprocal space. The band folding process in the Brillouin zone provides the key contribution to the observed overall reduction in the scattering losses and improving the overall robustness of the quasi-BIC resonances in the hybrid lattice. The research demonstrates a noteworthy increase in radiative Q factors within the hybrid lattice (H-qBIC), surpassing the uniform lattice (U-qBIC) by 3-fold enhancement at THz frequencies (see Fig. 1(e)). Further, the team observed a progressive enhancement in overall quality factors across distinct types of hybrid supercells (H-qBICs). A notable feature of the H-qBIC lattice is its remarkable enhancement in the radiative Q factors and immunity to its symmetry breaking. The numerical results show 16 times enhancement and high stability in their Q factors at a large value of asymmetry degree, and further validated by their experimental results. This stability hints at a saturation of radiative Q factors at larger asymmetries, ensuring that the Q factors remain robust and do not deteriorate due to fabrication imperfections or disorders.
In summary, this experiment highlights the potential of lattice engineering to enhance the robustness and efficiency of symmetry-protected BICs and opens new avenues for applications in hyperspectral sensing, where high-quality resonances are crucial. As the research community delves deeper into these lattice and symmetry architectures for fostering the potential of BICs, the possibilities for advanced photonics and optoelectronic devices will continue to expand, promising a future of enhanced performance and versatile photonic technologies.