【AIGC One Sentence Reading】:Bottom-up nanostructures offer a promising solution for efficient microLED fabrication and integration, enabling voltage and geometry-controlled multi-wavelength, high-speed light emission for nanoscale optoelectronics.
【AIGC Short Abstract】:The future of optoelectronics lies in small-area light sources, with microLEDs leading the way. Despite fabrication and integration hurdles, new solutions using bottom-up nanostructures show promise. Lan Fu's team has exhibited nanowire array LEDs, controlling multi-wavelength and high-speed operations through voltage and geometry adjustments, highlighting nanostructures' potential in nanoscale optoelectronics.
Note: This section is automatically generated by AI . The website and platform operators shall not be liable for any commercial or legal consequences arising from your use of AI generated content on this website. Please be aware of this.
To date, however, fabricating devices at micron and submicron scales is not straightforward. During typical top-down device fabrication of epilayers, the device mesa is defined using a reactive-ion etching step. This dry etch process severely damages the sidewalls of the device mesa, leaving a large number of crystalline defects and dangling bonds, which contribute to non-radiative recombination of charge carriers1, 2. These surface states degrade the light output, and hence efficiency, of ultra small optoelectronic devices, rendering them impractical for use. The damage caused by the etching process is especially disadvantageous in devices with a smaller mesa size, due to the increased ratio of surface-area-to-volume. Intensive research has been dedicated to healing the damage induced on the surface, through methods such as annealing, wet chemical etching and/or surface passivation, but these have been met with limited success3, 4.
Lately, micron-scale, or smaller, optoelectronic devices have emerged as a frontier technology, particularly micron-scale or smaller light emitting diodes (LEDs), known as microLEDs. These small-area devices are direct upgrades to their larger counterparts in areas such as displays or illumination, while they are also carving out their own unique niches in applications such as biology and communication.
This work encapsulates the many distinct advantages of bottom-up nanostructure-based devices over conventional top-down etched devices in the context of microLEDs. The major improvements in this work were all contingent on the epitaxy: from the regular and controlled nanowire morphology, which is an indicator of defect-free crystal epitaxy, to the excellent electrical and light output characteristics of finished devices. The flexibility of the emission from the nanostructure-based arrays, with nanostructure geometry, allows for the monolithic integration of multiple multi-wavelength LEDs for wavelength division multiplexing, demonstrated as a proof of concept by the authors of this work, as shown by the arrangement of multiple small micro-LED arrays to form the letters “ANU” in Fig. 1(f). The inset of this figure contains an infrared camera image of the EL emission from the nanowire array LED. The authors are seeking to further extend this idea by creating individually addressable pixels consisting of single nanowire, or small-area array LEDs.
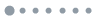
Figure 1.(a) Schematic of the nanowire cross-section top and profile view. (b) An SEM image of a nanowire array. (c) Schematic of the fabricated nanowire LED. (d) L-I-V curves for a representative nanowire LED. (e) Normalized voltage-dependent EL spectra. (f) SEM image of nanowire arrays arranged to form the letters “ANU”. The inset shows an infrared camera image of the EL emission from the nanowire array LEDs.
Recently, Prof. Lan Fu from the Australian National University, and their colleagues, published a work in Opto-Electronic Science, where they grew highly uniform p-i-n core-shell InGaAs/InP single quantum well (QW) nanowires, using metalorganic chemical vapor deposition (MOCVD), that were subsequently fabricated into microLED arrays12. A schematic representation of the nanowires is shown in Fig. 1(a). Uniform nanowire arrays with regular morphology were grown, and a scanning electron microscope (SEM) image is shown in Fig. 1(b). Due to variations in Indium incorporation along different facets, emission in the telecom wavelength range of 1.35 µm — 1.5 µm was measured from the nanowire arrays. The authors also noted a dependence of emission wavelength on the nanowire dimension and spacing — this phenomenon has been observed previously and is attributed to the effect of these parameters on the local supply of adatoms/reactants13, 14. Furthermore, by selecting for a low temperature, high V/III ratio growth regime, the authors have been able to minimize height differences between the different nanowires; thereby greatly simplifying the process to integrate the different nanowire arrays on a single substrate for multi-pixel applications.
To study device performance, the nanowire arrays were fabricated to form microLED devices. A schematic of the final device is shown in Fig. 1(c). The authors demonstrated nanowire array LEDs with rectifying characteristics, low turn-on voltage and an improvement in output power by over 6×, as compared to a similar previously reported device15. The current-voltage and light output curves are shown in Fig. 1(d). The electroluminescence (EL) observed from the device also shows the presence of multiple and broad peaks, at approximately 1.35 µm and 1.5 µm, which is expected due to the complex geometry of the nanowire structure, as well as the myriad current injection paths available for carriers to follow and recombine in. Injection dependent spectra show that the emission blueshifts towards shorter emission wavelengths at higher biases, potentially enabling voltage-tunable multi-wavelength operation. Simulations performed by the authors were able to identify the emission at 1.5 µm to originate from the relatively larger volume radial QW which dominates emission, while the shorter peak at 1.35 µm originated in the smaller volume axial QW. At high biases, the band-filling effect starts to become dominant in both the axial and radial QWs, pushing the peak emission to shorter wavelengths. The normalized EL spectra at different biases are plotted in Fig. 1(e). The broad EL emission peak with a FWHM of ~286 nm is well suited for applications such as bio-sensing and optical coherence tomography.
The concern of etch damage can be completely sidestepped using a bottom-up process to grow device mesas. The process of selective area growth (SAG) involves patterning vias to the substrate on a mask layer, and the patterned substrate is then loaded for growth. The growth conditions are tuned such that epitaxy only occurs within the openings defined. This results in the growth of nano(micro)structures, having dimensions and shapes matched exactly to those defined while patterning the substrate5, 6. Furthermore, these nanostructures would not need to be exposed to any dry etching process to define device mesas, thereby preventing the formation of the surface defects associated with that process. These advantages are necessary to attain a high efficiency for any (sub)micron-scale device. Nanostructures can also be grown on non-native substrates, potentially opening up more new applications7. In addition, previous research has highlighted numerous other benefits of nanostructures over conventional epilayer-based devices, including a nearly defect-free crystal, enhanced dopant incorporation and reduced strain8, 9. SAG can also be used to grow nanostructures in specific arrangements, such as a photonic crystal, which can be harnessed for uses such as in directional LEDs or ultra-small surface-emitting lasers10, 11.
With microLED technology being intensively studied at the present, the authors showcased the numerous benefits of SAG to form bottom-up nanostructures for micro and nanoscale optoelectronics. While the authors have used the InGaAs/InP material system, other work has demonstrated similar enhancements to selective area grown microLEDs fabricated from other material systems – this underlines the incredible versatility of SAG and bottom-up approaches to drive improvements in nanoscale light sources operating at any wavelength.
Optical communication and photonic circuits both require high speed operation. To evaluate the ultrafast operation of the devices, the authors used time-resolved photoluminescence spectroscopy to measure the minority carrier lifetimes. The measured lifetime of only ~309 ps is nearly 4× lower than bulk InP nanowires, indicating efficient carrier recombination. The measured lifetime sets an upper limit of ~3 GHz for the modulation frequency. Time-resolved electroluminescence also showed that the nanowire array LED could be modulated at GHz frequencies. The authors suggested that optimization of the ITO p-contact and removal of the InP substrate could greatly reduce the parasitic capacitance and series resistance of the device, effectively overcoming the modulation frequency limitations imposed by the RC constants.