1. INTRODUCTION
Donor–acceptor organic solar cells (OSCs) have achieved tremendous progress since the reports of bulk-heterojunction (BHJ) organic solar cells [1]. Early in its development, fullerenes and their derivatives led to an era of OSCs due to their excellent electron mobility, large electron acceptability, and isotropy of charge transport [2–4]. However, the inherent huge energy losses and weak photon-harvesting capacities of fullerenes limited their further applications [5,6]. Compared with fullerene acceptors, the non-fullerene acceptors (NFAs) usually have strong absorption in the near-infrared region (NIR), and their energy levels can be easily adjusted to match the donors, which have been proved to promote the power conversion efficiencies (PCEs) of OSCs [7–12]. Driven by the vigorous development of materials science, the PCEs of single-junction OSCs have recently exceeded 17% [13–21]. Despite the impressive achievements made by NFAs, the single NFA usually suffers from incomplete absorption of the solar spectrum and relatively low charge mobility compared with perovskite components [22]. In order to make full use of the advantages of fullerene and NFAs, multicomponent blending is recommended to construct multiple devices. The incorporation of fullerene and NFAs into the BHJ layer provides a promising approach to improve the photovoltaic performance of OSCs.
The recent rapid progress of OSC materials, especially NFAs with variable molecular structures and tunable energy levels, enables a large material pool for realizing the potential of multicomponent BHJ solar cells [23–29]. Li et al. [30] earlier reported an NFA-based binary system BDB-T:ITIC mixed with binary fullerenes as acceptor additives. Consequently, the optical band gaps of the NFA-blended films were reduced and the carrier transport processes were enhanced. As a result, the open-circuit voltage (), the short-circuit current-density (), and the fill factor (FF) were simultaneously improved, promoting an ultimate PCE up to 12.8%. Bi et al.[31] developed an individual nanostructure optimized quaternary system based on two donors and two acceptors. High crystallinity DR3TBDTT was dispersed in PTB7-Th to enhance the domain purity, while was used as phase modifier to promote favorable FOIC packing. Thus, the nanoscale morphologies of the donors and the acceptors were optimized individually, which helped to increase carrier mobilities and suppress monomolecular recombination, thereby contributing to a champion PCE of 13.51%. Recent work from Ma et al. [32] proposed an efficient quaternary system combining a basic binary blend including fullerene and NFA. The third component, Br-ITIC, was added to maximize the photon harvesting, and was used as the fourth component to optimize the molecular arrangement and phase separation of the active layer, leading to a PCE of 16.8%. Therefore, multicomponent blending is an effective strategy to promote the PCEs of OSCs. However, quaternary or ternary blending has rarely been applied in large-area photovoltaic modules.
In this work, a quaternary blending strategy was used in the fabrication of small-area photovoltaic devices and large-area photovoltaic modules. We have explored a quaternary system, including one polymer donor PM6, one fullerene acceptor , and two NFAs Y6 and ITIC. The was added as the third component in the PM6:Y6 system to optimize the charge transport. The ITIC was further added as the fourth component to maximize the photon harvesting. As a result, a champion PCE of 16.71% was achieved in the quaternary device after an optimization of photovoltaic parameters. Furthermore, a certified PCE of 12.36% was achieved for the large-area () module, which is one of the highest efficiencies for inverted organic solar modules to date. The excellent photovoltaic performance demonstrates the great potential of using multicomponent organic solar modules for practical applications.
Sign up for Photonics Research TOC Get the latest issue of Advanced Photonics delivered right to you!Sign up now
2. EXPERIMENT
A. Materials
The PM6, Y6, ITIC, and were purchased from Solarmer Materials Inc. The ZnO precursor solution was prepared by dissolving zinc acetate dehydrate and ethanolamine in the solution of 2-methoxyethanol with a concentration of 0.5 mol/L.
B. Device Fabrication
The multiple OSCs were constructed with indium tin oxide (ITO)/ZnO/active . For small-area device fabrication, the ZnO precursor solution was spin-coated on the oxygen plasma-pretreated ITO at 3000 r/min and baked at 200°C for 1 h in air. PM6, Y6, ITIC, and were dissolved in chloroform with different mass ratios. The mass concentration of the polymer was kept at . Chloronaphthalene (0.8%) was added to optimize the phase separation. The blend solutions were then spin-coated onto the ZnO layer at an optimized speed of 2400 r/min, obtaining the thickness of , and were subsequently annealed at 50°C for 10 min. Finally, a 10 nm thick layer was deposited as the anode interlayer by thermal evaporation under less than , followed by deposition of 80 nm of Ag as the top electrode.
For large-area module fabrication, the modules were fabricated with a similar architecture to the small-area OSCs. The patterned ITO-coated glass substrates ( with P1 scribe) were spin-coated with a ZnO precursor solution at 3000 r/min and baked at 200°C for 1 h in air. The quaternary blend solution () was prepared with a polymer weight concentration of . Chloronaphthalene (0.8%) was added as additive. The quaternary solution was spin-coated on the ZnO layer at 5000 r/min in atmosphere, and the films were subsequently thermally annealed at 50°C for 10 min. Then a 10 nm thick layer was deposited as the anode interlayer by thermal evaporation under less than . Finally, 80 nm of Ag was thermally evaporated under less than . The series connection is realized with the typical P1-P3 laser (532 nm, 10 ns) patterning method, as shown later in this paper, and the geometrical filling factor is 95.5%.
C. Characterization
The density-voltage (J-V) curves were measured by a Keithley 2400 source meter under AM 1.5 G light () from an SAN-EI electric solar simulator. The light intensity was calibrated using a standard silicon solar cell. The typical small-device area of is defined by a shadow mask. For the large-area module, the total illumination area is , and the external quantum efficiency (EQE) was recorded by QE-R from Enli Technology. The UV-vis absorption spectra were measured by a Agilent Carry 5000 UV-vis spectrometer. Atomic force microscopy (AFM) images of the sample surfaces were obtained on a Cypher S atomic force microscope.
3. RESULTS AND DISCUSSION
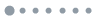
Figure 1.(a) Chemical structures and (b) normalized absorption spectra of PM6, Y6, ITIC, and . (c) J-V curves and (d) EQE spectra of the multiple OSCs.
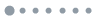
Figure 2.AFM height images and values of the multiple OSCs. equals (a) 1:1.4:0:0, (b) 1:1.2:0:0.2, (c) 1:1.15:0.05:0.2, (d) 1:1.1:0.1:0.2, (e) 1:0.6:0.6:0.2, and (f) 1:0:1.2:0.2.
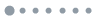
Figure 3. curves of the multiple OSCs.
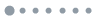
Figure 4.(a) Device architecture, (b) realistic image, and (c) J-V curve of the large-area organic photovoltaic module. (d) The cellphone charged by the large-area organic photovoltaic module (see Visualization 1).
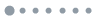
Figure 5.Independent certification result of the large-area module based on the (1:1.15:0.05:0.2) device from the Shanghai Institute of Microsystem and Information Technology, Chinese Academy of Sciences, confirming a high PCE of 12.36% (Certificate No. 19TR120402) ().
4. CONCLUSION
In summary, we successfully fabricated a series of high-performance multiple OSCs. Fullerene and NFA (namely, and ITIC) were sequentially added to the binary system to optimize the photovoltaic properties of the multiple blend films. With the strategy of integrating the advantages of multiple components, an optimized PCE up to 16.71% was obtained. Based on the exploration of quaternary devices, a large-area photovoltaic module was manufactured with a PCE of 13.25% (certified as 12.36%). The excellent performance of photovoltaic devices and large-area modules proves the synergy of multiple components, paving a way to the commercial application of the high-performance photovoltaic cells.
APPENDIX A
The main text presents the photovoltaic parameters of the large-area module measured in house. Here shows the independent certification result of the large-area module (Fig.?5). A certified PCE of 12.36% was obtained.