1. Introduction
Magnetic semiconductors (MSs) are promising materials for spintronic applications, which would be useful for non-volatile and low-power-consumption electronic devices[1−5]. The ideal MSs are expected to at least satisfy the following requirements: first, their Curie temperature should be higher than room temperature (300 K); and second, both p-type and n-type MSs could be realized. Mn-based Ⅲ−Ⅴ MSs have been intensively investigated, such as p-type (In,Mn)As[6−10] and (Ga,Mn)As[11−15], while the maximum TC are still much lower than room temperature (90 K in (In,Mn)As[10], 200 K in (Ga,Mn)As[13]). However, the effort made by previous research could not realize a MS satisfying these two requirements, until new results were found in Fe-doped Ⅲ−Ⅴ MSs. Both n- and p-type MSs could be achieved by doping Fe into Ⅲ−Ⅴ host semiconductors, such as p-type (Ga,Fe)Sb (with maximum TC = 400 K)[16−20] and n-type (In,Fe)Sb (with maximum TC = 385 K)[21−23]. Moreover, plenty of recent research has focused on the microscopic origin of the ferromagnetism of (Ga,Fe)Sb, revealing the intrinsic ferromagnetism of (Ga,Fe)Sb films [19, 20, 24−26].
The magnetic anisotropy of (Ga,Fe)Sb (7.6 × 103 erg/cm3 at xFe = 29.8%) is much smaller than the typical value of (Ga,Mn)As (3.6 × 104 erg/cm3)[27], which makes it difficult to be utilized for practical applications. In this work, we co-doped Ni with Fe into GaSb host semiconductor and achieved enhanced magnetic anisotropy of Ga1-x-yFexNiySb films than that of (Ga,Fe)Sb. As a result, the Ku of Ga1-x-yFexNiySb is negligible at y = 1.7% but increases to 3.8 × 105 erg/cm3 at y = 6.1%, while the Curie temperature of samples was maintained above 300 K. Additionally, the hole mobility of Ga1-x-yFexNiySb films reaches at 31.3 cm2/(V∙s) at x = 23.7%, y = 1.7%, which is much higher than the mobility of Ga1-xFexSb at x = 25.2% (µ = 6.2 cm2/(V∙s)).
2. Experiments
The Ga1-x-yFexNiySb films were grown on semi-insulating GaAs(001) substrates by low temperature molecular beam epitaxy (LT-MBE), a schematic sample structure is shown in Fig. 1(a). At first, we grew a 150-nm-thick GaAs buffer layer to obtain a smooth GaAs surface at 550 °C. Then, a 5-nm-thick AlSb was deposited at 470 °C as a seed layer, following with a 100-nm-thick Al0.9Ga0.1Sb buffer layer grown at 470 °C to relax the strain induced by the lattice mismatch between Ga1-x-yFexNiySb and GaAs. Compared with AlSb, Al0.9Ga0.1Sb buffer achieves a smoother surface, while the buffer layer keeps insulating due to the low concentration of Ga. After that, a Ga1-x-yFexNiySb layer of 100 nm with almost the same Fe concentration x and different Ni concentration y was grown at the growth rate of 0.4 μm/h at 250 °C. Finally, a 2-nm-thick GaSb cap layer was grown at 250 °C to prevent oxidation of underlying Ga1-x-yFexNiySb layer. We grew two series of Ga1-x-yFexNiySb samples, A1−A4 and B1−B4, and the sample information is listed in Table 1. We changed x of samples A1−A4 (x = 25.2%−29.8%) and kept y = 0, to investigate the magnetic anisotropy and hole mobility of (Ga,Fe)Sb. Samples B1−B4 with nearly constant x (x ≈ 24%) and changed y (y = 1.7%−6.1%) were used to study the y dependence of the magnetic and electronic properties of Ga1-x-yFexNiySb samples. In order to manifest the evolution of Ku and µ of Ga1-x-yFexNiySb, the x of samples A1−A4 was roughly equal to the sum of x (B1−B4) and y (B1−B4), respectively.
![(Color online) (a) Schematic layer structure of Ga1-x-yFexNiySb samples. (b) The RHEED pattern of an undoped GaSb sample. (c)−(f) RHEED patterns taken along the [110] azimuth after the growth of Ga1-x-yFexNiySb layers for samples B1−B4 (x ≈ 24%, y = 1.7%−6.1%).](/Images/icon/loading.gif)
Figure 1.(Color online) (a) Schematic layer structure of Ga1-x-yFexNiySb samples. (b) The RHEED pattern of an undoped GaSb sample. (c)−(f) RHEED patterns taken along the [110] azimuth after the growth of Ga1-x-yFexNiySb layers for samples B1−B4 (x ≈ 24%, y = 1.7%−6.1%).

Table 1. Curie temperature TC, hole mobility μ at 10 K, saturation magnetization MS and anisotropy constant Ku of Ga1-x-yFexNiySb films with different Fe concentration x and Ni concentration y.
Table 1. Curie temperature TC, hole mobility μ at 10 K, saturation magnetization MS and anisotropy constant Ku of Ga1-x-yFexNiySb films with different Fe concentration x and Ni concentration y.
Sample | x (%) | y (%) | TC (K) | μ (cm2/(V∙s)) | MS (emu/cc) | Ku (erg/cc) |
---|
A1 | 25.2 | 0 | 337 | 6.2 | 142 | −1.8 × 103 | A2 | 26.9 | 0 | 351 | 3.4 | 144 | 1.2 × 103 | A3 | 28.0 | 0 | 362 | 1.9 | 147 | 4.5 ×103 | A4 | 29.8 | 0 | 375 | 1.1 | 151 | 7.6 ×103 | B1 | 23.7 | 1.7 | 319 | 31.3 | 148 | −2.1 × 103 | B2 | 24.2 | 2.8 | 336 | 17.9 | 152 | 1.4 × 105 | B3 | 23.9 | 4.5 | 343 | 7.5 | 159 | 2.3 × 105 | B4 | 24.3 | 6.1 | 354 | 4.6 | 165 | 3.8 × 105 |
|
The reflection high-energy electron diffraction (RHEED) was used to observe the surface morphology and crystallinity of the samples. The lattice constant of Ga1-x-yFexNiySb layers was investigated by X-ray diffraction (XRD). Scanning transmission electron microscopy (STEM) and selective area electron diffraction (SAED) were employed to characterize the crystal structure and impurity concentration of the Ga1-x-yFexNiySb layers. The magnetic and electronic properties of Ga1-x-yFexNiySb were separately characterized by superconducting quantum interference device (SQUID) magnetometer and physical property measurement system (PPMS).
3. Results and discussion
3.1. Crystalline properties
The RHEED pattern of an undoped GaSb film grown with the same condition as the Ga1-x-yFexNiySb samples is displayed in Fig. 1(b). Figs. 1(c)−1(f) present the RHEED patterns of samples B1−B4 taken along the [] axis after the growth of Ga1-x-yFexNiySb layers. It is obvious that the RHEED patterns of Ga1-x-yFexNiySb layers are streaky with surface reconstruction of 1 × 3, which is similar with that of GaSb. The results imply that Ga1-x-yFexNiySb layers grown by LT-MBE keep the zinc-blende crystal structure.
XRD spectra of samples A1−A4 are shown in Fig. 2(a), while that of samples B1−B4 are shown in Fig. 2(b), which are both performed using the Cu-Kα radiation (wave length λ = 0.15406 nm). No phases other than Al0.9Ga0.1Sb and Ga1-x-yFexNiySb can be observed in the XRD spectra, and in particular there are no Fe−Sb, Ni−Sb intermetallic compounds or metal clusters. The concentration of Fe and Ni is preliminary determined by a series of energy dispersive spectra, which are measured repeatedly at different positions in Ga1-x-yFexNiySb layer. According to the dependence between the Fe (Ni) doping concentration and Fe/Ga (Ni/Fe) flux ratio, the x and y could be further confirmed, as listed in Table 1. The lattice constant of (Ga,Fe)Sb and Ga1-x-yFexNiySb could be given by a1 = (1−x)aGaSb + xaFeSb and a2 = (0.76−y)aGaSb + 0.24aFeSb + yaNiSb. Here, aGaSb, aFeSb and aNiSb are the lattice constant of GaSb, hypothetical zinc-blende FeSb and NiSb, respectively, which are estimated to be 0.60648 nm (aGaSb), 0.55027 nm (aFeSb) and 0.58233 nm (aNiSb) as plotted in Figs. 2(c) and 2(d). The results are consistent with previous research[16].
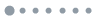
Figure 2.(Color online) (a) XRD spectra of Ga1-x-yFexNiySb samples A1−A4 (x = 25.2%−29.8%, y = 0). (b) XRD spectra of samples B1−B4 (x ≈ 24%, y = 1.7%−6.1%). (c) x dependence of the lattice constant of A1−A4. (d) y dependence of the lattice constant of B1−B4.
The crystal structure and impurity concentration of samples A1−A4 and B1−B4 were characterized by STEM and SAED. Fig. 3(a) shows the STEM lattice image of sample B4 taken along the [110] axis, showing the clear interface between Ga1-x-yFexNiySb layer and buffer layer. Through the enlarged picture area marked by red rectangle in Fig. 3(a), the crystal structure of Ga1-x-yFexNiySb layer is displayed more clearly in Fig. 3(c). Fig. 3(b) shows the SAED pattern of Ga1-x-yFexNiySb layer of representative sample B4 (x = 24.3%, y = 6.1%). Due to the nonuniform Fe distribution in sample B4 with high doping concentration[17], the shaded areas in Fig. 3(c) probably are caused by the influence of the strong ferromagnetism. Generally, there are no visible defects in Ga1-x-yFexNiySb layer and no phases other than Ga1-x-yFexNiySb.
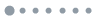
Figure 3.(Color online) (a) The cross-sectional STEM image of typical Ga1-x-yFexNiySb sample B4 (x = 24.3%, y = 6.1%). (b) SAED pattern of the Ga1-x-yFexNiySb layers in sample B4. (c) The area marked by red rectangles shown in Fig. 3(a).
3.2. Magnetic properties
A SQUID magnetometer was used to investigate the magnetic properties of Ga1-x-yFexNiySb samples. Fig. 4 shows the magnetization hysteresis curves (M−H) of samples B1−B4 (x ≈ 24%, y = 1.7%−6.1%) measured at 10 K, with the magnetic field H being applied along the [110] and [001] axes. Clear hysteresis curves are observed and shown in Fig. 4, which demonstrate the presence of ferromagnetic order of Ga1-x-yFexNiySb layers. For the samples with y = 1.7%, the in-plane magnetization and perpendicular magnetization show similar characteristics, suggesting small magnetic anisotropy in this sample. For the samples with y = 2.8%−6.1%, the difference between in-plane and perpendicular magnetization is remarkable. The perpendicular magnetization saturates at a much smaller magnetic field than the in-plane magnetization, indicating that the easy axis of magnetization is perpendicular to the plane and there is a strong magnetic anisotropy in these samples. As shown in Fig. 4, the perpendicular saturation magnetic fields of samples B1−B4 are marked by the blue arrows. In addition, the saturation magnetization MS values increase as y increases, which are 148, 152, 159 and 165 emu/cm3 at y = 1.7, 2.8, 4.5 and 6.1%. The magnetic anisotropy constant could be estimated by , where M⊥ and M|| represent the perpendicular and in-plane magnetization, respectively. Here, the saturation field HS means the intersection of the in-plane and perpendicular M−H loop, which is marked by the red arrows in Fig. 4. It is found that the Ku is negligible for y = 1.7% but increases to 2.3 × 105 erg/cm3 for y = 4.5% and 3.8 × 105 erg/cm3 for y = 6.1%, suggesting the improvement of Ni doping to the magnetic anisotropy of Ga1-x-yFexNiySb. Additionally, the magnetization hysteresis curves of samples A1−A4 (x = 25.2%−29.8%, y = 0) show similar characteristics with that of sample B1, of which the Ku can also be neglected. The results imply that Ni doping can effectively improve the magnetic anisotropy of Ga1-x-yFexNiySb.
![(Color online) Magnetic hysteresis curves (M−H) measured at 10 K of samples B1−B4 (x ≈ 24%, y = 1.7%−6.1%) with a magnetic field applied along the [001] axis and the [110] axis. The blue and red arrows in (a)−(d) signify the perpendicular saturation magnetic fields and the positions of HS.](/Images/icon/loading.gif)
Figure 4.(Color online) Magnetic hysteresis curves (M−H) measured at 10 K of samples B1−B4 (x ≈ 24%, y = 1.7%−6.1%) with a magnetic field applied along the [001] axis and the [110] axis. The blue and red arrows in (a)−(d) signify the perpendicular saturation magnetic fields and the positions of HS.
3.3. Transport properties
Hall data of samples B1−B4 was collected to characterize the magnetic transport properties of the Ga1-x-yFexNiySb films. Samples B1−B4 were fabricated into Hall bars with a size of 200 × 40 μm2. The hole mobility of Ga1-x-yFexNiySb is determined by , where the Rxy, Rxx , l and w are the anomalous Hall resistance, the longitudinal Hall resistance measured at zero-field, and the length and width between the electrodes of Hall bar, respectively. In order to accurately determine the hole mobility of Ga1-x-yFexNiySb, the influence of AHE should be excluded. Thus, we measured Rxy at low temperature (10 K) and calculated the ΔRxy/ΔB at high magnetic field (up to 16 T). As shown in Fig. 5, we measured the magnetic field dependence of Hall resistance curves (RHall−H) of samples B1−B4 (x ≈ 24%, y = 1.7%−6.1%) at 10 K. As plotted in the insets of Fig. 5, the obvious hystereses at low temperature reveal that RHall is dominated by the AHE in the low magnetic field range. Moreover, the red dashed lines in Fig. 5 almost coincide with the RHall−H curves at high magnetic fields, implying that the magnetization is nearly saturated. The mobility of Ga1-x-yFexNiySb samples B1−B4 (x ≈ 24%) is listed in Table 1, which decrease from 31.3 cm2/(V∙s) at y = 1.7% to 4.6 cm2/(V∙s) at y = 6.1%. As for samples A1−A4 (y = 0), µ values also decline from 6.2 cm2/(V∙s) at x = 25.2% to 1.1 cm2/(V∙s) at x = 29.8%. Both Ni doping and Fe doping would reduce the hole mobility of Ga1-x-yFexNiySb, however, samples co-doped with Fe and Ni exhibit higher mobility than that doped with Fe solely.
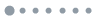
Figure 5.(Color online) The magnetic field dependence of Hall resistance curves (RHall−H) of the samples B1−B4 (x ≈ 24%, y = 1.7%−6.1%) measured at 10 K.
The Curie temperatures of samples B1−B4 (x ≈ 24%) are estimated by the Arrott plots of the RHall−H curves, which rise from 319 K (y = 1.7%) up to 354 K (y = 6.1%). With regard to samples A1−A4, the TC values of these samples also increase from 337 K at x = 25.2% to 375 K at x = 29.8%, demonstrating similar impurity concentration dependence characteristics. The effects of Ni doping on mobility and TC behave similar but weaker than that of Fe doping.
4. Conclusion
In summary, we co-doped Ni with Fe into GaSb host semiconductor by using LT-MBE, and achieved enhanced magnetic anisotropy and hole mobility of Ga1-x-yFexNiySb films compared with that of (Ga,Fe)Sb. XRD and STEM results reveal that there are no second phases in Ga1-x-yFexNiySb films. Moreover, we obtained the anisotropy constant Ku (up to 3.8 × 105 erg/cm3) and hole mobility µ (up to 31.3 cm2/(V∙s)) in Ga1-x-yFexNiySb films, which are much higher than that of (Ga,Fe)Sb (with the maximum Ku = 7.6 × 103 erg/cm3 and maximum µ = 6.2 cm2/(V∙s)). Our results indicate that Ga1-x-yFexNiySb is a promising magnetic semiconductor for spintronic applications.