1. INTRODUCTION
Since its discovery in 2004, carbon nanodots (C-dots) have gained much attention due to their fantastic advantages of low toxicity, biocompatibility, water solubility, and excellent photostability [1–5]. Owing to the excellent fluorescence properties of C-dots in an aqueous solution, various applications based on C-dots have been investigated, such as bioimaging probe [6,7], metal ion detection [8], and photocatalysis [9,10]. However, the performance of optical devices based on C-dots is highly limited for its aggregation-induced quenching effects when they are dried into films or powders. Recently, a template-supported strategy has been used to investigate the optical property of C-dots in solid state through embedding C-dots into tremendous host matrices [11,12]. Among them, zeolite has emerged as an ideal template to synthesize C-dots for its various identical nanopore systems and high thermal stability [13–16]. The solid state C-dots@zeolite shows specific optical properties, such as multicenter photoluminescence [17], long lifetime, and phosphorescence [18,19]. Despite the large advance in C-dot synthesis and application, the nonlinear optical saturable absorption properties and its application in ultrafast pulsed laser generation are seldom explored [20]. It is noted that ultrafast pulsed lasers with mode-locking have wide applications in optical telecommunication, biomedical diagnostics, and laser precision manufacturing [21–23].
A nonlinear optical element called a saturable absorber (SA) was widely used to generate ultrafast pulsed fiber lasers. In the past decades, a semiconducting saturable absorber mirror (SESAM) has been developed as a promising SA to generate ultrafast fiber lasers [24]. However, the SESAM’s fabrication process is complicated and expensive, greatly limiting its further application. Recently, low-dimensional materials, such as carbon nanotubes [25–28], graphene [29–32], graphene oxide [33–35], topological insulators [36–40], black phosphorus [41], and layered metal dichalcogenides have been investigated as SAs to generate ultrafast pulsed fiber lasers [42–44]. However, there are a few drawbacks associated with these materials for ultrafast photonics devices. For example, many of these SA materials are composited with polymer, which have a drawback of poor dispersity and are inclined to aggregation, inducing the instability of photonics devices. Besides, these SA materials can operate at only a relatively low pump power for the unavoidable thermal effect on the polymer at a high incident laser power. It is therefore of great importance to find new nonlinear materials with low cost, good stability, and a high optical damage threshold in broadband laser operation.
In this work, we present the fabrication of C-dots with a uniform size by pyrolysis of an n-propylamine (NPA) that is confined in zeolite Linde Type A (LTA) topology. The C-dots@LTA exhibits nonlinear saturable absorption properties at 1.5 μm and 1 μm. By inserting the C-dots@LTA SA into the fiber laser cavity, mode-locked ultrafast pulsed fiber lasers with good long-term operation stability can be achieved at 1.5 μm and 1 μm, respectively. Our work demonstrates that C-dots@zeolite could be a new composite material for broadband nonlinear optics and its application in ultrafast photonics.
Sign up for Photonics Research TOC Get the latest issue of Advanced Photonics delivered right to you!Sign up now
2. EXPERIMENTAL SECTION
A. Preparation and Characterization of Materials
Zeolite LTA single crystals were hydrothermally synthesized in a reaction system of . Typically, was added in a solution of with distilled water and stirred for 4 h. Then, the NPA was added into the gel solution and stirred for another 2 h. The as-obtained gel was sealed into a teflon-lined stainless-steel autoclave and heated to 190°C for 30 h. The as-synthesized sample was washed with distilled water and dried at 80°C for 3 h. After that, the as-synthesized LTA crystals were placed into a quartz boat and heated from room temperature to 400°C with a heating rate of 5°C/min under vacuum condition. Then, the C-dots@LTA composite was obtained by maintaining 400°C for 3 h. The isolated C-dots were obtained by removing the LTA template. Typically, the as-synthesized C-dots@LTA sample was immersed in hot 3 mol/L NaOH for 2 h and followed by concentrated HCl under ultrasonic treatment. Then, the dark-colored solution was washed with distilled water for six times, and the insoluble samples were centrifugally separated from the solution.
Powder X-ray diffraction (XRD) patterns were collected with a Bruker D8 system. The morphology of the LTA was investigated using a scanning electron microscope (SEM, JEOL Co., Model: JSM-6049LA). The single crystal X-ray diffractometry (Xcalibur, Sapphire3, Gemini Ultra) was carried out for characterizing the structure of crystal. Transmission electron microscopy (TEM) measurements were executed on FEI TECNAI G2 60-300 equipment at an acceleration voltage of 300 kV. The Raman spectrum was obtained using a Horiba JY Labram HR800 with the 514.5 nm laser excitation.
B. Experimental Setup of Fiber Lasers
The laser setup of an erbium-doped fiber laser (EDFL) and ytterbium-doped fiber laser (YDFL) is shown below. A 980 nm laser diode (LD) was used as the pump power source and transmitted into the cavity through a 980/1550 nm wavelength-division multiplexing (WDM) coupler (980/1060 nm for YDFL). An optical isolator (ISO) was placed into the ring laser cavity to ensure the unidirectional propagation of the laser. A polarization controller (PC) was used to adjust the polarization state of light in the cavity. One single LTA zeolite crystal hosting C-dots (C-dots@LTA) was picked onto a fiber optic jumper to form a fiber-compatible SA by using an extra-thin copper wire with the help of an optical microscope and then was inserted into the laser cavity to induce the mode-locked fiber laser operation. The thickness of the C-dots SA is about 100 μm according to the cubic morphology of LTA zeolite. A 10:90 output coupler was used to output the laser. A 1.5-m-long heavily erbium-doped fiber (LIEKKI Er80-8/125, cutoff wavelength: , absorption at 1530 nm) with a group velocity dispersion (GVD) of at 1550 nm and a 2-m-long heavily ytterbium-doped fiber (LIEKKI Yb1200-4/125, cutoff wavelength: , 1200 dB/m absorption at 976 nm) with GVD of at 1060 nm were used as the gain medium, respectively. For the EDFL, the other fibers are standard single-mode fiber with a length of 5 m, and the GVD is . As for the YDFL, the other fibers are single-mode fiber (HI 1060) with a length of 4.1 m, and the GVD is . The total laser cavity length is about 6.5 and 6.1 m for EDFL and YDFL, respectively. Thus, the cavity dispersions of EDFL at 1550 nm and YDFL at 1060 nm were estimated at and , respectively. The output lasers were analyzed by using an optical spectrum analyzer with a resolution of 0.02 nm, a 20 GHz high-speed oscilloscope (Tektronix MSO 72004C) together with a 45 GHz photo-detector (New Focus 1014), and a power meter, respectively. The corresponding pulse duration was also measured by an autocorrelator (APE, Pulsecheck SM1200).
3. EXPERIMENTAL RESULTS
Figure 1 illustrates the method to prepare the C-dots@LTA composite with nonlinear saturable absorption properties by in situ carbonization of NPA into C-dots in the confined space of zeolite LTA.
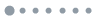
Figure 1.Schematic of the synthesis process of C-dots@LTA composite material.
First, the LTA crystals were hydrothermally synthesized by using NPA as the structure-directing agent. The powder XRD profile of the as-synthesized sample matches well with the corresponding diffraction results for the simulated XRD pattern of LTA and the other reported XRD pattern of LTA [45,46], indicating phase-pure LTA synthesis with high crystallinity [Fig. 2(a)]. Figure 2(b) shows the corresponding SEM image of zeolite LTA crystals, which have a well-shaped cubic morphology; its average size is about 100 μm. The space group of as-synthesized LTA is Pm3m symmetry with lattice constants (1 Å = 0.1 nm), , and their unit volume is , as shown in Fig. 2(c).
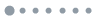
Figure 2.(a) Experimental (upper) and simulated (lower) XRD patterns of LTA. (b) SEM image of as-synthesized LTA crystals. (c) Framework structure of the LTA single crystal retrieved from single crystal XRD data.
Then, the C-dots@LTA composite is obtained by pyrolysis of NPA@LTA at 400°C for 3 h. The TEM characterization of isolated C-dots confirms the narrow size distribution with average size of 2 nm, as shown in Fig. 3(a). The high-resolution TEM image reveals their lattice spacing to be 0.21 nm, which is consistent with the lattice spacing of the (100) plane of graphene [Fig. 3(b)] [47]. The powder XRD pattern of the isolated C-dots shows a broad peak at 26°, corresponding to the characteristic graphitic peak [Fig. 3(c)] [47,48]. The typical D-band () and G-band () can be clearly observed in the Raman spectrum [Fig. 3(d)], agreeing well with other reported C-dots [49,50].
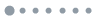
Figure 3.(a) TEM image (inset: size distribution), (b) high-resolution TEM image, (c) powder XRD pattern, (d) Raman spectrum of as-synthesized C-dots.
A balanced twin detector measurement system was used to investigate the nonlinear optical characteristics of the as-synthesized C-dots@LTA composite, as shown in Fig. 4(b). The evolution of the absorption ratio was obtained by increasing the pump peak density with home-made pulsed fiber lasers at 1550 nm and 1050 nm, respectively. The data for normalized absorption at 1550 nm and 1050 nm can be well fitted according to a simple two-level saturable model [25,29]. Based on the fitting results, the modulation depth and saturable intensity at 1550 nm are determined to be 7.7% and , respectively [Fig. 4(c)]. Similarly, the modulation depth and saturable intensity at 1050 nm are determined to be 6.8% and , respectively [Fig. 4(d)]. The modulation depth of the tested C-dots@LTA composite is comparable to other carbon materials [25,29,51], but the normalized nonabsorption loss is larger. This is tolerable for fiber lasers with a relatively large single roundtrip gain coefficient [25,52]. In our case, the contributions to the nonabsorption loss may include scattering and refraction from the surface of LTA zeolite and linear coupling loss between fiber ends. These results clearly demonstrate that the C-dots@LTA composite could be used as an SA for generating ultrafast pulsed lasers in a broad wavelength band.
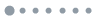
Figure 4.(a) Schematic diagram of the erbium-doped and ytterbium-doped fiber laser. (b) The setup of a balanced twin-detector measurement. The normalized absorption of the C-dots@LTA SA as a function of pump pulse peak intensity with excitation wavelength of (c) 1550 nm and (d) 1050 nm, respectively: dots, measured data; red line, fitting to the data.
To explore the applicability of the as-synthesized C-dots@LTA in ultrafast pulsed fiber laser generation, we constructed two all-fiber ring cavities by using EDF and YDF as the gain medium, respectively [Fig. 4(a)]. Before inserting the C-dots@LTA SA into the laser cavity, the laser was insensitive to the polarization state and always worked in the continuous wave mode with an increase in the pump power, which can exclude the nonlinear polarization rotation effect in the laser cavity. As expected, the mode-locking operation of the EDFL was obtained by putting the C-dots@LTA SA into the EDFL cavity when pump power was beyond the mode-locked laser threshold of 150 mW. Figure 5(a) shows the emission spectrum of above mode-locked pulsed fiber laser. The spectrum with two Kelly bands is a typical feature of soliton mode-locked lasers [53]. The operating central wavelength is about 1564.9 nm with a 3 dB spectral bandwidth of 4.84 nm. Figure 5(b) displays a 23 MHz repetition rate of the mode-locked pulse train (period ), which matches well with the laser cavity length (6.5 m). The pulse width is about 609 fs, assuming a pulse profile, as shown in Fig. 5(c). Figure 5(d) shows the radio frequency (RF) spectrum of the mode-locked laser output after optical-to-electrical conversion using a fast photodiode. The signal-to-noise ratio (SNR) is over 59 dB, indicating a good mode-locking stability. Figure 5(e) shows the output power of the mode-locked EDFL as a function of the pump power. As the pump power increases from 150 to 310 mW, the output power increases almost linearly from 0.56 to 6.02 mW, resulting in a slope efficiency of 3.4%. Figure 5(f) shows the long-term stability of the mode-locked laser output spectrum measured at 6 h intervals over 1 day. We can clearly find that the mode-locked EDFL is very stable as its central wavelength and the 3 dB bandwidth are kept almost unchanged.
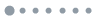
Figure 5.Output characteristic of the EDFL operated in mode-locking state. (a) Emission spectrum. (b) Pulse train. (c) Single pulse profile. (d) RF spectrum. (e) Output power as a function of the pump power. (f) The output spectrum measured every 6 h showing long-term stability of the mode-locking soliton state.
Similarly, the mode-locked YDFL can be obtained by inserting the C-dots@LTA SA into the YDFL cavity when the pump power is beyond the mode-locked laser threshold of 130 mW. The operating central wavelength is about 1051.3 nm with a 3 dB spectral bandwidth of 0.74 nm, as shown in Fig. 6(a). The spectrum with steep edges is a typical shape of dissipative solitons [54]. Figure 6(b) displays a 24.6 MHz repetition rate of the mode-locked pulse train (period ), which matches well with the laser cavity length (6.1 m). The pulse width is about 966 ps, as shown in Fig. 6(c). It is widely known that mode-locked Yb-doped fiber lasers with SAs are mostly dissipative solitons with large chirp, a natural result of the balance among the cavity loss, gain, nonlinearity, and dispersion. Normally, the time bandwidth product (TBP) is widely used to estimate the degree of chirp in pulsed fiber lasers [28,55,56]. The TBP is calculated as 194 in an Yb-doped fiber laser, which means the dissipative solitons are strongly chirped, inducing the expanded pulse duration. The SNR of the RF spectrum is over 68.6 dB, indicating a good mode-locking stability [Fig. 6(d)]. Figure 6(e) shows the output power of the mode-locked YDFL as a function of the pump power. As the pump power increases from 130 to 290 mW, the output power increases almost linearly from 0.46 to 7.42 mW, resulting in a slope efficiency of 4.4%. The mode-locked YDFL is also very stable as its central wavelength and 3 dB bandwidth are kept almost unchanged [Fig. 6(f)]. To estimate the damage threshold of the SA, we increased the pump power to 650 mW (the maximum of the 980 nm LD that we used) and kept it for 2 h. After that, we decreased the pump power from 650 to 0 mW, and both of the stable mode-locked Er-doped and Yb-doped fiber lasers were obtained again in their stable pump power range, which is 150 to 310 mW and 130 to 290 mW, respectively. This means the C-dots@LTA SA is not damaged at the pump power of 650 mW. Thus, the optical damage threshold of the SA is over 650 mW.
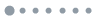
Figure 6.Output characteristic of the YDFL operated in mode-locking state. (a) Emission spectrum. (b) Pulse train. (c) Single pulse profile. (d) RF spectrum. (e) Output power as a function of the pump power. (f) The output spectrum measured every 6 h showing long-term stability of the mode-locking dissipative soliton state.
Table 1 summarizes data of some reports about mode-locked fiber lasers with a different carbon-based SA. It shows that our C-dots@LTA SA is comparable to other carbon-based SAs, especially for the recently reported novel carbon-based SA [51,57,58]. Moreover, there are some advantages by using C-dots@LTA as the SA to generate ultrafast pulsed fiber lasers. (1) The stability of pulsed fiber lasers is improved, which can be maintained over several months. The LTA zeolite channels offer a solid nanospace to fix C-dots with well dispersion and prevent the aggregation of C-dots. (2) The photonics device is easy to handle. The pulsed fiber lasers can be generated by putting one single LTA zeolite crystal that hosts C-dots between two fiber connectors to form a fiber-compatible SA. (3) The C-dots@LTA SA has a high optical damage threshold, which is over 650 mW. (4) The C-dots@LTA is a cost-effective broadband SA, which can be fabricated at a relatively low carbonization temperature of 400°C and can be used for generating Er-doped and Yb-doped pulsed fiber lasers.

Table 1. Typical Mode-Locked Fiber Lasers with Different Carbon-Based SAs
Table 1. Typical Mode-Locked Fiber Lasers with Different Carbon-Based SAs
SA Type | Wavelength (nm) | Pulse Duration (ps) | SNR (dB) | Slope Efficiency | Reference | Carbon nanotubes | 1518–1558 | 0.706 | 70 | | [25] | 1025.5 | 0.175 | 63 | | [28] | Graphene | 1565 | 0.756 | 65 | 3% | [29] | 1069.8 | 580 | 70 | | [55] | Graphene oxide | 1559.56 | 0.582 | 56 | 2.6% | [57] | Mesoporous carbon | 1036 | 445 | | 2.58% | [51] | Graphdiyne | 1564.7 | 0.734 | 41.77 | 1% | [58] | C-dots@LTA | 1564.9 | 0.609 | 59 | 3.4% | Our work | 1051.3 | 966 | 68.6 | 4.4% | Our work |
|
4. CONCLUSION
Zeolite LTA single crystals were used as a template for the fabrication of C-dots with a uniform size. The nonlinear optical property of as-synthesized C-dots@LTA composite was investigated, and the application of C-dots@LTA as a new broadband saturable absorber for ultrafast pulsed fiber laser generation was achieved. By inserting the C-dots@LTA SA into the fiber laser cavity, mode-locking operation in EDFL and YDFL with long-term stability was achieved. The mode-locked soliton pulses in EDFL have a central wavelength of 1564.9 nm with the pulse width of 609 fs and repetition rate of 23 MHz. As to the YDFL, the mode-locked dissipative soliton pulses have a central wavelength of 1051.3 nm with a pulse width of 966 ps and repetition rate of 24.6 MHz. Our work reveals the C-dots@zeolite is a promising SA material for ultrafast pulsed fiber laser generation in a broad wavelength band.