Within this context, researchers led by Castellanos-Gomez and Xie proposed an all-dry, inexpensive fabrication method based on abrasion of WS2 (tungsten disulfide) on paper to fabricate photoconductive detectors covering a bandwidth from near infrared to ultraviolet10. Their maximum responsivity (a measure of the conversion efficiency of the input light to the output current) is significantly higher than those of similar devices in the literature. The main drawback is that the devices are negatively impacted by exposure to ambient oxygen, suggesting the need of an encapsulating layer to improve their performance.
A comparison with other photodetectors in the literature shows superior responsivity performance, both with respect to devices on flexible substrates based on other photoconductive materials (such as WSe2), and also to other WS2 devices based on more traditional, solvent-free deposition methods such as sputtering or chemical vapor deposition (CVD).
The fabrication process is remarkable in its use of readily available materials and simple and effective techniques, which has been previously developed by Castellanos-Gomez’s group11, 12. Specifically, a cotton swab is used to transfer WS2 powder on a standard printer paper substrate, essentially exfoliating single flakes from the van der Waals material into a relatively thick (around 20 μm) layer (Fig. 1(a)). The rough paper surface ensures a larger effective area (and a consequent higher responsivity). The device geometry (around 2 mm) is defined by protecting the paper with removable adhesive tape. The electrical contacts are similarly created with a soft graphite pencil, simultaneously defining the active channel length (of around 250 μm at least). Alternatively, a narrower channel (45 μm) is obtained by evaporating thin (100 nm) gold contacts through a shadow mask. Both fabrication paths avoid liquid solvents and high temperature processing.
Systematic characterization of several identical devices showed a moderate dispersion of the responsivities, which is not unexpected because of the inherently percolative nature of conduction paths through the randomly arranged WS2 flakes, and which clearly represents a limiting aspect with respect to other deposition techniques as CVD, which, as a point of strength, allows better material uniformity.
As a demonstration of the potential of the fabrication technology, authors have exploited the detectors in a proof-of-concept spectrometer (Fig. 1(b)), showing remarkable correspondence with the spectra determined with a commercial silicon photodiode, which demonstrates the possibility of fabricating cost-effective and high-performance electronic/optoelectronic devices on biofriendly substrates as the paper.
The primary figure of merit of photoconductive detectors is the responsivity (measured in AW-1), defined as the ratio between the device photocurrent Iph and the corresponding incident light power P:
In the last decades, several applications based on the pervasiveness of inexpensive, versatile, environmentally friendly electronic devices have been envisioned, ranging from mobile healthcare to entertainment, to wearable systems (body trackers, smart textiles, etc.), to countless others. These applications will be based on the convergence of several new technologies, such as flexible substrates and new versatile high-performance materials. Among the former, paper is an extremely appealing choice, because of its biodegradability and negligible cost, allowing the development of systems, which can have a reduced environmental impact at the end of their life cycle1, 2. From the technological point of view, the main challenges concerned with device fabrication on paper are related to the high surface roughness, the limited thermal budget (around 120 °C) and the poor stability (especially with respect to humidity), which requires purposely tailored fabrication techniques. 2D materials can represent an enabling technology for paper-based electronics and optoelectronics systems, due to their outstanding properties for apparently any class of applications, and naturally suited for flexible applications3-7. However, integration of 2D materials on paper substrates presents important challenges that have not been yet satisfactorily addressed. The most common transfer techniques of 2D materials on flexible substrates, such as liquid-assisted layer transfer or printing, requires exposure of the paper to various solvents and/or drying steps, both of which quickly degrade the substrate: for this reason, dry transfer techniques are of great interest8, 9.
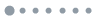
Figure 1.(a) Sketch of the fabrication process. (b) Schematic of the set-up for the testing of the proof-of-concept spectrometer.10
Another important figure of merit, which has been widely investigated and compared against the results available in the literature is the response time, able to provide relevant information regarding the speed of the photodetector. The measured values are of the order of seconds, in line with other photodetectors fabricated through solution processed techniques (e.g., inkjet printing, drop casting, dip coating, etc.), but way larger (several order of magnitude) as compared to CVD or sputtering: this is clearly the current limitation of the proposed technique, which can hamper its exploitation in high-speed optoelectronic systems. It is anyway undoubtful that the adopted technique is probably the best (and cheapest) for a really fast prototyping of optoelectronic devices, and, while it cannot be used in all those applications where fast response of the photodetectors is required (smaller than 10–3 s), it can be exploited whenever the bandwidth of the signal is limited. This is, for example, the case for biosignals, where the proposed technology could enable a wide range of disposable electronic applications13.
To ensure consistency in the characterization, responsivity measurements were performed in vacuum. In this condition, the WS2 devices shown very good linearity (i.e., a responsivity essentially independent from the input power). In general, the responsivity is expected to be an increasing function of the bias voltage, and the devices under test showed a dramatic increase moving from 5 to 35 V, up to a responsivity of around 0.27 A/W in graphene/WS2 devices at 617 nm (red-orange) light. Predictably, Au/WS2 devices, owing to their shorter length, presented a much higher responsivity than the equivalent graphene/WS2 ones.
The fabricated devices exhibit a reversible and repeatable resistance modulation under bending of the paper substrate, a fact that can be attributed to flake-on-flake sliding and consequent change of the overlap area. The devices are also sensitive to oxygen: their dark current (i.e., the current under no illumination) reversibly increase around fivefold if the device is moved from ambient air to oxygen-deprived air or to vacuum.