1 Introduction
With the rapid development of wireless electronic technology, an increasing number of electronic sensors are being installed on airborne platforms to achieve various functions. Traditional multi-antenna layout schemes suffer from severe electromagnetic compatibility issues. Considering practical engineering constraints such as platform functionality, size, weight, and power consumption, the traditional multi-antenna aperture is gradually being replaced by ultra-wideband comprehensive radio frequency (RF) apertures. On the other hand, with the rapid advancement of stealth technology in materials, antennas are becoming the main scattering source for carrier platforms. At the same time, as a special scattering target compared to conventional targets, antennas need to minimize their own scattering while ensuring good radiation performance [1]. Additionally, there is very limited installation space reserved on airborne platforms which are continuously evolving towards flatness. Therefore, conformal design between antennas and airborne platforms is required to enhance stealth and aerodynamic performance [2,3]. Hence, airborne antennas need to meet performance requirements such as ultra-low profile, ultra-wideband capability, easy conformance, low scattering, and omnidirectional radiation.
The traditional method to achieve miniaturization and wideband characteristics in omnidirectional antennas is by loading the antenna structure or bending the current flow [4–8]. In Ref. [4], top-loading and short-circuit loading techniques were used. Top-loading provided a capacitive load to increase the effective height of the antenna, while short-circuit loading further extended the current path. These measures facilitated low-profile miniaturization of the antenna, reducing its profile height to 0.085 times the wavelength at nearly 4 times the frequency bandwidth. Reference [5] bent the normal of a traditional inverted triangular monopole antenna based on a single cone antenna design, which elongated the current path and achieved smoother impedance variation. This resulted in a miniaturized design with lateral dimensions of 0.2 times the wavelength and profile height of 0.1 times wavelength, enabling bandwidth close to 10 times the frequency range. In addition to top-loading and short-circuit loading, reference [6] employed dielectric loading by attaching high-permeability material on both sides of a metal wire, achieving miniaturization with a profile height as low as 0.07 times the wavelength within a working bandwidth four times higher than the frequency range. Reference [7] simplified traditional radiation cones into symmetrically placed four curved metal arms that extended the current path and introduced multiple resonance points for both miniaturization and wider bandwidth achievement. The operating bandwidth for this antenna was from 1.6 GHz to 4 GHz with a profile height of approximately 0.057 times the wavelength.
Although these antennas have achieved certain levels of miniaturization and wideband characteristics, their profiles are still relatively high compared to conformal antennas used in next-generation airborne platforms. Thus, further breakthroughs are needed to achieve lower profile heights while keeping wideband performance.
Generally, the bandwidth of a thick dipole is wider compared to a thin dipole. The antenna bandwidth can be further expanded by adding sleeves on top of a monopole antenna [9]. In Ref. [10], two additional sleeve rings were added around a conical antenna, effectively expanding the impedance bandwidth of the antenna and achieving a bandwidth of 5.3 octaves. The profile height was 0.07 times the low-frequency wavelength. A similar monopole-like omnidirectional vertical polarization radiation mode can also be achieved using two coupled current loops. In Ref. [11], an antenna structure was formed using two short-circuit diamond-shaped bent metal surfaces and a rectangular metal plate at the top. Each diamond-shaped bent metal surface, along with its mirrored element, can equivalently form a current loop. This structure significantly reduces the lowest operating frequency and enables miniaturization of the antenna, with a profile height of only 0.033 times the low-frequency wavelength with an operation bandwidth of 4 octaves.
In addition to using traditional monopole structures for achieving omnidirectional vertical polarization radiation, it can also be achieved using a circular ring array. The profile height of the circular ring slot antenna proposed in Ref. [12] is only 5 mm, which can be directly embedded into the skin of the aircraft body. However, this antenna has a limited bandwidth and can only operate in the range of 2.3 GHz–2.5 GHz. In order to broaden the operating bandwidth of the circular ring array, reference [13] proposes bending a two-dimensional planar long slot array into a cylindrical array and adding a hybrid ground plane consisting of the ferrite/EBG (electromagnetic band gap) structure to enhance gain while maintaining wideband characteristics and achieving horizontal omnidirectional radiation. This antenna can operate in the range of 150 MHz–600 MHz but has a relatively high-profile height of 0.39 times the wavelength at low frequencies. The aforementioned antennas also face limitations in terms of bandwidth and larger size at lower frequency bands.
Currently, whether through structural loading or curved current bending, the miniaturization design on traditional monopole structures, or utilizing circular ring array structures for achieving ultra-wideband low-profile antennas with vertical polarization radiation, there are still challenges regarding the insufficient bandwidth width, high profile, and difficulty expanding at lower frequencies. Based on the excellent ultra-wideband and low-profile characteristics possessed by tightly coupled arrays as well as traditional low-profile loading techniques, this paper presents a design for an extremely low-profile tightly coupled ultra-wideband vertically polarized omnidirectional antenna with a profile height equivalent to only 0.047 times the wavelength at low frequencies.
2 Design of antenna element structures
The main objective of this article is to design an ultra-wideband, low-profile, vertically polarized omnidirectional antenna for airborne antenna applications. To achieve both ultra-wideband and low-profile characteristics, a tightly coupled antenna is selected as the element. By utilizing a circular array with equal-amplitude and in-phase feeding, the tightly coupled planar array can be transformed into a conformal array along the circular ring. As shown in Fig. 1, N identical antenna elements with vertical polarization are uniformly distributed along the circular ring. Through properly designing baluns and power dividers, equal-amplitude and in-phase feeding are applied to each element to achieve ultra-wideband omnidirectional radiation.
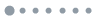
Figure 1.Schematic diagram of omnidirectional conformal ring array based on tightly coupled antenna element. ( denates the amplitude excitation of the n-th port, ra represents the distance from the centroid of the regular octagon to the center of the antenna element.)
The antenna element depicted in Fig. 2 (a) employs a long slot-type tightly coupled dipole element. By arranging the planar array into a circular array, the original H-plane (long slot direction) of the planar array aligns with the formation direction of the circular array, while the E-plane direction corresponds to the vertical direction after forming the circular array and determines the antenna’s profile height. To achieve an extremely low profile, it is necessary to truncate in the E-plane direction instead of using infinitely periodic units and minimize the number of elements. Considering that two elements can be connected with a power divider at the rear ends to reduce the input impedance of tightly coupled elements and facilitate impedance matching, two elements are employed in the E-plane.
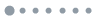
Figure 2.Structure diagram of tightly coupled long slot element: (a) traditional element and (b) element with short-circuit wall. (h denotes the height between the ground and long slot element.)
The structure shown in Fig. 2 (a) lacks a strong coupling environment due to only 2 elements in the E-plane, resulting in current truncation at the edges, which leads to strong edge-generated reflection waves and impedance mismatch within the operating bandwidth. To suppress edge effects, as shown in Fig. 2 (b), a short-circuit wall is adopted, where the short-circuit metal piece can be equivalent to an electric wall. According to the principle of mirroring, it can approximate adding mirror elements at the edges and partially compensate for the lack of strong coupling conditions. Additionally, on the short-circuit surface, suitable gaps are added with lumped resistors loading to partially absorb edge reflection waves.
The simulation results presented in Fig. 3 illustrate the standing wave ratio of the planar element model in a one-dimensional periodic environment after the use of short-circuit processing and resistive loading. It is evident that a peak still exists in the standing wave ratio curve due to ground reflection at higher frequencies. To address this issue, a resistive frequency-selective surface is introduced between the long slot element and the metal ground. Fig. 4 presents the unit structure of the resistive frequency-selective, along with its S parameters and absorption rate obtained through simulation in a two-dimensional periodic environment. The absorption rate can exceed 30% notably at frequencies where the wave ratio reaches its peak, effectively absorbing energy that is radiated towards the ground.
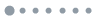
Figure 3.Standing wave ratio of the planar element model after the use of short-circuit processing and resistive loading (fl denotes the lowest operating frequency in the working band).
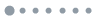
Figure 4.Final tightly coupled long slot element model.
Fig. 5 shows the final model of the tightly coupled ultra-wideband antenna element, which mainly includes a long slot dipole, feeding balun and power divider network, resistive plane, resistive frequency-selective layer, and metal ground. The specific dimensions of the element are $0.055{\lambda _l} \times 0.046{\lambda _l} \times 0.046{\lambda _l}$ (where λl is the low-frequency wavelength). Fig. 6 presents a comparison of the standing wave ratio curves between the tightly coupled long slot element and a connected array element with the same dimensions. It can be observed that this tightly coupled long slot element exhibits good impedance matching performance and can be used for the conformal circular array design.
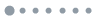
Figure 5.Resistive frequency selective unit and its simulation results.
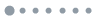
Figure 6.Comparison of the standing wave ratio curves between the tightly coupled long slot element and a connected array element.
3 Design of omnidirectional ring array structure
3.1 Improved element structure design for circular arrays
To achieve vertically polarized omnidirectional radiation in the horizontal plane, the tightly coupled elements need to be arranged in a conformal circular array. Firstly, the impact of the number of elements in the circular array on the array factor is investigated. Fig. 7 shows the radiation patterns corresponding to different numbers of elements, with a fixed circular array radius. It can be observed that as the number of elements increases, the circularity of the radiation pattern gradually improves due to the improved beam overlap of 3 dB. Considering the need for good circularity and the simplicity of fabrication, the final number of elements is selected as 8.
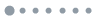
Figure 7.Impact of different element numbers on the azimuth radiation pattern of the circular array. (φ is the azimuth angle, N is the antenna element number, f denotes the highest operating frequency, and a represents the radius of the circular array.)
Additionally, as the conformal circular arrangement makes the area of the dipole layer, the resistive frequency-selective layer, and the metal ground decrease sequentially, modifying the element structure as shown in Fig. 8 (a) is required. However, the change in the element structure inevitably affects its electrical performance. To compensate for the performance degradation caused by these structural changes, two improvements are employed for the element structure, as shown in Fig. 8 (b): Firstly, the shorting plane connecting the dipole to the ground plane is extended outward to increase the lateral electrical length; secondly, the metallic ground plane is bent and extended to increase its effective area.
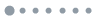
Figure 8.Tightly coupled antenna element structure for circular conformal array: (a) before improvement and (b) after improvement.
3.2 Circular arrays design
Based on the antenna element structure shown in Fig. 8, a final omnidirectional circular array can be obtained (see Fig. 9). Specifically, the process involves first arranging an 8-element linear array (Array I) uniformly in a circular configuration to form Array II. Then, the element structure is modified to create Array III. Finally, improvements are made to the modified element structure, resulting in the final Array IV, which is an 8-element tightly coupled omnidirectional circular array. This antenna array has an extremely low profile, with a height of only 0.047 times the low-frequency wavelength and a maximum lateral diameter of 0.19 times the low-frequency wavelength, demonstrating ultra-low-profile characteristics.
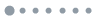
Figure 9.Schematic diagram of the construction process of the omnidirectional circular conformal array.
4 Results and analysis
4.1 Active standing wave ratio characteristics
The actual antenna prototype is presented in Fig. 10. Due to the fact that the omnidirectional conformal ring array has eight feeding ports, the active voltage standing wave ratio (VSWR) at each antenna port cannot directly be measured by a two-port vector network analyzer. Thus, it is necessary to measure all the scattering parameters for each port of the array with respect to the other ports. Subsequently, the active VSWR at the m-th port, denoted by Active_Sm, can be calculated using the following formula [14]:
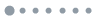
Figure 10.Actual antenna prototype of the omnidirectional conformal ring array.
$ {\text{Active}}\_{S_m} = \left| {\sum\limits_{n = 1}^N {{S_{m{\mathrm{,}}\;n}}\frac{{{a_n}}}{{{a_m}}}} } \right| $ (1)
where Sm, n represents the measured S parameters between the m-th element and the n-th element; αm and αn are the feeding magnitude and phase of the m-th port and the n-th port, respectively.
The measured active VSWRs for each port of the omnidirectional conformal ring array are shown in Fig. 11. It can be observed that under the condition of active VSWRs less than 3, the designed omnidirectional conformal ring array has an impedance bandwidth close to 12∶1, demonstrating significant ultra-wideband characteristics. This capability can meet the multifunctional application requirements for airborne antennas.
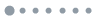
Figure 11.Measured active VSWR for each port of the omnidirectional conformal ring array.
4.2 Array radiation characteristics
The gain pattern of the omnidirectional conformal ring array is measured in a microwave anechoic chamber. During the testing process, a one-to-eight power divider is used to combine the array into a single port to obtain the array’s omnidirectional gain pattern. It should be noted that the final results need to account for the insertion loss introduced by the power divider.
The measured gain patterns of the circular array at some typical frequency points in the azimuth plane are shown in Fig. 12. As a comparison, the corresponding simulation results are also provided in Fig. 12. Clearly, the measured and simulated results are in good agreement. The out-of-roundness of the gain patterns can be controlled within 3 dB across the entire operation bandwidth, exhibiting a good omnidirectional radiation characteristic. The measurement errors of the gain patterns are mainly caused by three factors: 1) systematic error of gain measurement, 2) manufacturing error of the antenna prototype, and 3) main beam alignment error.
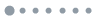
Figure 12.Measured and simulated gain patterns of the omnidirectional conformal ring array at some typical frequency points: (a) 2fl , (b) 4fl , and (c) 10fl .
5 Conclusions
This paper proposes an airborne ultra-wideband circular ring conformal array antenna based on the typical tightly coupled ultra-wideband long slot element and traditional miniaturization methods of omnidirectional antennas. This antenna exhibits a profile height of only 0.047 wavelengths at low frequencies, with a maximum lateral diameter of 0.19 wavelengths at low frequencies, enabling it to cover a working bandwidth of 12∶1. It possesses the advantages of ultra-wideband with an extremely low profile, while maintaining good out-of-roundness (less than 3 dB) for the azimuthal vertical polarization pattern across the operating frequency range.
To summarize, the proposed tightly coupled ultra-wideband low-profile omnidirectional circular ring conformal array antenna exhibits good performances such as ultra-wideband, low profile, vertical polarization, and high-gain omnidirectional radiation, showing promising potential in airborne antenna applications.
Disclosures
The authors declare no conflicts of interest.