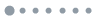
Figure 1.(Color online) (a) Traditional design and (b) all-fused ring design toward D-A-type fused-ring electron acceptors. (c) Molecular structures of Typical AFRAs.
In contrast, AFRAs with marginally larger bandgaps can serve as visible opaque and NIR transmitting (VONIRT) materials because of their exceptionally broad absorption spectra within the visible spectrum and significant transparency in the NIR range. Wang et al. created a series of AFRAs referred to as FM-F and FM-OCH3[16], which have wide absorption spectra spanning from 400 to 800 nm with the full width at half maximum of 299 nm. Importantly, both FM-F and FM-OCH3 demonstrated outstanding photostability due to their fully fused-ring structures. The VONIRT optical filter based on FM-F and FM-OCH3 effectively blocked all visible light while permitting over 80% transmittance of NIR light. This selective extraction of NIR signals from complex environments has numerous applications in real-world contexts related to NIR, such as security, forensic science, and anti-counterfeiting measures.
In conclusion, the advancement of AFRAs is essential for improving the thermal, chemical, and photostability of OSCs and OPDs, even though this field is still in its infancy. In this research highlight, we have emphasized the benefits and applications of recent AFRAs. However, the PCEs of OSCs utilizing AFRAs remain constrained. Thus, additional efforts to optimize the optoelectronic properties of these materials are needed to boost photovoltaic performance.
Wang et al. developed a 14-fused-ring AFRA named FM2, which exhibited an exceptionally small optical bandgap of 1.22 eV, an onset absorption wavelength at 1013 nm, and an increased electron mobility of 7.0 × 10−4 cm2∙V−1∙s−1[1]. Due to its stable molecular conformation and minimal conformational disorder, the FM2 thin film demonstrated a reduced trap density of 5.55 × 1016 cm−3. Consequently, the FM2-based OPD exhibited a decreased level of dark current density (Jd) of 22.01 × 10−10 A∙cm−2 at 0 V, a Dsh* exceeding 1013 Jones within the wavelength spectrum of 400 to 1000 nm, along with a maximum specific detectivity of 4.65 × 1013 Jones at a wavelength of 880 nm. These findings rank among the highest reported for self-powered NIR OPDs and suggest that AFRA holds great potential for developing high-performance NIR sensing devices. Recently, Wang et al. re introduced a fully-fluorinated AFRA named FM-8F, which has eight fluorine atoms attached to its terminals[15]. This fluorination approach resulted in a lowered lowest unoccupied molecular orbital (LUMO) energy level, a redshifted absorption spectrum, and significantly improved charge separation. These features contributed to enhanced photoresponsivity, achieving a specific detectivity of approximately 1012 Jones in the 550−1000 nm wavelength range, compared to around 1011 Jones for the non-fluorinated AFRA (FM-0F) based OPD device.
Near-infrared (NIR) responsive compounds with narrow bandgaps play a crucial role in enhancing the photovoltaic efficiency of organic solar cells (OSCs) by effectively capturing high-energy NIR photons, as well as improving the NIR sensitivity of organic photodetectors (OPDs) through NIR light detection[1, 2]. The design principle based on electron-donating and accepting (D−A) structures has led to the development of numerous A−D−A or A−DA’D−A type NIR responsive molecules, such as ITIC and Y6. These compounds are extensively used in high-efficiency OSCs that achieve power conversion efficiencies (PCE) reaching 20%[3−5], and they also find application in highly sensitive NIR OPDs with detectivity (D*) values exceeding 1013 Jones. Typically, these molecules consist of conjugated heteroaromatic rings serving as central D/DA’D units, paired with two terminal As derived from 2-(3-oxo-2,3-dihydro-1H-inden-1-ylidene)malononitrile (INCN), which are interconnected via two vinyl linkages (Fig. 1(a)). However, the exocyclic double bonds generated through the kinetically reversible Knoevenagel condensation reaction are prone to photooxidation and decomposition under alkaline conditions. This vulnerability results in poor stability when exposed to light or basic environments, making them less suitable for practical applications[6, 7]. Consequently, it is both important and challenging to create an organic molecule that possesses a narrow bandgap while simultaneously exhibiting good stability and outstanding efficiency.
Although there have been significant advancements in PCE, challenges related to stability and cost continue to hinder the commercialization of OSCs. As previously noted, AFRAs exhibit inherently superior photostability and chemical stability when compared to leading acceptors featuring INCN-type terminals. To tackle the cost issue, Zhu and his team have introduced two novel AFRAs, designated F11 and F13 (Fig. 1(c))[12, 13], and have developed an extensive array of synthetic methods for the large-scale production (~10 g) of F11 and F13 in a notably brief period. As a result, the synthesis process for F11 and F13 has shown both low complexity and minimal costs compared to other reported INCN-type nonfullerene acceptors, even rivaling the commercial photovoltaic material P3HT. The introduction of an electron-withdrawing BT unit as the central core led to a more red-shifted absorption spectrum, enhanced J-aggregation, and a three-dimensional honeycomb-like packing structure in F11 and F13. Consequently, devices based on D18:F13 achieved a high PCE of 13% (compared to 9.51% and 11.2% for D18:ITYM and D18:F11, respectively). OSCs utilizing F11 and F13 exhibited enhanced photostability, which can be attributed to the intrinsic stability of AFRAs. This is supported by the significantly lower burn-in losses when compared to OSCs that use ITIC and Y6. Additionally, AFRA promoted stacking between the donor's benzene ring and the acceptor's end group, facilitating a direct excitation mechanism through borrowing oscillator strength. This enabled multiple charge transfer processes to occur simultaneously within the same complex, accounting for the high performance of this OSC device. Later, Zhu et al.[14] detailed the development of all-small-molecule ternary OSCs that utilized IDT-IC (medium bandgap) and F13 (narrow bandgap) as non-fullerene molecular acceptors, in combination with C1-CN (wide bandgap) serving as the molecular donor. The highest reported PCE achieved was 15.54%, marking it as the leading efficiency for OSCs based on AFRAs to date.
Beyond their application in OSCs, AFRAs have also been recognized as active materials for organic photodetectors (OPDs). Their fully fixed conformation and resulting low conformational disorder enable AFRAs to effectively reduce dark current and improve the performance of OPDs. In 2022, Zhu et al. developed a narrow-bandgap AFRA (PDTTYM, see Fig. 1(a)) that features a thin-film absorption onset at 1020 nm and created four PDTTYM-based OPDs[2]. These OPDs demonstrated cutoff frequencies nearing 100 kHz and shot-noise-limited specific detectivity (Dsh*) exceeding 1012 Jones in the range of 780 to 950 nm under zero bias, which is comparable to that of commercial silicon-based photodetectors.
Recently, Xiaozhang Zhu group introduced the concept of "all-fused-ring electron acceptor (AFRA)" (Fig. 1(b))[8]. These AFRAs possess entirely fixed structures and lack exocyclic double bonds, which provides them with distinct advantages such as excellent planarity and rigidity, improved electron delocalization, and reduced molecular vibration and rotation. As a result, they exhibit remarkable chemical stability and photostability. AFRAs have been widely utilized in organic solar cells (OSCs) and organic photodetectors (OPDs), demonstrating superior photostability compared to devices that utilize ITIC or Y6. Therefore, we have compiled recent developments regarding AFRAs along with their applications.
In 2021, Zhu and colleagues introduced the AFRA concept and successfully synthesized a nine-fused-ring compound named ITYM (Fig. 1(b)), which is defined as 2,2′-(7,7,15,15-tetrahexyl-7,15-dihydro-sindaceno[1,2-b:5,6-b]diindeno[1,2-d]thiophene-2,10(2H)-diylidene)dimalononitrile)[8]. Single-crystal X-ray diffraction studies indicated that ITYM exhibited robust one-dimensional π−π stacking at both ends of its molecular structure, enhancing electron transport capabilities. When compared to conventional carbon-bridged non-fullerene acceptors, ITYM demonstrated remarkable thermal stability as well as chemical and photostability while achieving an efficiency nearing 10%. To further improve photovoltaic performance, Wang and his team introduced a 10-fused-ring AFRA named FM1 in 2022 (Fig. 1(c)). The chemical structure of FM1 is described as follows: 2,2′-(8-(2-butyloctyl)-16,17-dioctyl-16,17-dihydro-5H-indeno[1″,2″:4′,5′]thieno[2′,3′:4,5]pyrrolo[3,2-g]indeno[1′,2′:4,5]thieno [3,2-b][1,2,3]triazolo[4,5-e]indole-5,11(8H)-diylidene)dimalononitrile[9]. FM1 demonstrated an impressive electron mobility of 6.0 × 10−4 cm2∙V−1∙s−1 along with an optical bandgap of 1.50 eV and a maximum absorption wavelength at 769 nm. The organic solar cell (OSC) based on the FM1:D18 blend achieved a power conversion efficiency (PCE) of 10.8%. Notably, thanks to its excellent photostability and chemical stability properties of FM1, the OSC maintained remarkable device stability with only a 9% reduction in PCE after being subjected to simulated sunlight for 16 h. By increasing the conjugation of the D fragments and improving the electron-deficient characteristics of the A fragments, it is feasible to lower the optical bandgap of FM1. In 2023, Wang and colleagues created two 12-fused-ring AFRAs, named FM3 and FM4 (Fig. 1(c), derivatives of FM1), which exhibited reduced optical bandgaps of 1.44 and 1.33 eV, respectively[10]. Thanks to their fully fused-ring structures, both FM3 and FM4 demonstrated remarkable stability in terms of photoresponse, thermal conditions, and chemical interactions. The OSC based on the FM4:D18 blend achieved a power conversion efficiency (PCE) of 11.4%. Notably, this OSC maintained approximately 74% of its original PCE after being stored in an air environment for 456 h, whereas only about 53% was retained for the Y6:D18-based OSC. Utilizing a strategy of extending conjugation, Cui and colleagues introduced three AFRAs: DPT-T, DPT-TT, and DPP-T (Fig. 1(c)) to explore how different directions of π-conjugation affect their physicochemical properties, photovoltaic performance, and stability[11]. The variant DPT-TT, which features horizontally extended conjugation, exhibited a redshifted absorption spectrum along with an increased absorption coefficient, elevated energy levels, and enhanced crystallinity when compared to both DPT-T and DPP-T. When blended with PTQ10 as the donor material, the PTQ10:DPT-TT blend film showed improved phase separation between donor and acceptor components, more balanced charge carrier mobility, greater efficiency in exciton dissociation, and reduced recombination rates relative to the blends involving PTQ10:DPT-T or PTQ10:DPP-T. Consequently, the device utilizing DPT-TT achieved a significantly higher power conversion efficiency (PCE) of 12.14%, surpassing those based on DPT-T (PCE = 9.71%) and DPP-T (PCE = 6.58%). Additionally, all three devices displayed superior chemical stability as well as thermal and light soaking resilience compared to Y6-based devices−underscoring the attractive stability characteristics of all-fused-ring materials for use as acceptors in organic solar cells (OSCs).