1 Introduction
Nowadays, the millimeter wave (MMW) with a typical frequency range of 26.5 GHz–300.0 GHz is widely used in 5G and future 6G communications systems, due to its excellent characteristics of broadband and easy to miniaturize. As an essential component in the fields of communications, radar, medical imaging, wireless sensing, etc., MMW power detectors are gaining increasingly interest [1–4] and have rapidly advanced in the areas including the integrated circuit (IC) and modular system design, medical diagnostics, and security screening [5–8].
The earliest radio frequency (RF) power detector was primarily based on traditional analog circuit technologies, such as thermocouples and diode detectors [9]. These methods are simple, but the working frequency range is narrow and the achievable accuracy is low.
With the development of IC technology, the emergence of microwave ICs facilitated the miniaturization and integration of power detection modules [10], which endows such devices more diverse functions such as reflection coefficient measurement and temperature monitoring [11]. To enhance the accuracy and flexibility of detection, digital signal processing was also applied [12].
As the communications systems continued to evolve towards higher frequency bands and higher data rates, developing advanced power detection modules is becoming more and more urgent [13,14]. However, for high-frequency RF communications, especially MMW and terahertz bands, the realization of high-performance power detection modules with simple design is still challenging. Because the unique characteristics of high-frequency bands, communications environments, integration requirements, and emerging application needs should be taken into consideration during design. Advanced methods in circuit design and signal processing as well as novel materials are also necessary to ensure high-performance power detection in complex high-frequency communications environments.
Furthermore, higher frequency communications also necessitate the miniaturization, integration, and temperature stability of broadband power detection modules [15,16]. Such miniaturized MMW power detection module not only eases to integrate, thus satisfying the needs of numerous wireless communications devices and radar sensors, but also can effectively reduce the power consumption, thereby extending the battery lifespan and eliminating the limitation of power supplies. In addition, the miniaturization of MMW power detection modules can effectively reduce the number of components and manufacturing costs, which is helpful in large-scale production and further development of MMW technologies.
Currently, most systems for power detection adopt a two-chip or multi-chip structure [13]. With such a structure, a broad frequency band of 10 MHz–40 GHz can be detected by the power devices manufactured by Tektronix, Rod Schwartz, Agilent, and other companies. The absorption detector used in Ref. [17], which is designed by United Monolithlic Semiconductors based on the principle of diode detectors, can operate in the frequency band of 5 GHz–44 GHz. A 10 MHz–26.5 GHz detector chip is adopted in Ref. [18]. It is produced by Aglient and realized by the voltage comparator, analog-to-digital converter, and digital signal processor. Even though the power detection can be realized by quantifying the output voltage of the detector, such a split structure cannot be integrated into a small module.
This paper utilizes the low-barrier Schottky diode as the detection device for modeling and simulation and employs a comparator for threshold output. It designs and implements a power detection module which can operate in the frequency range of 26.5 GHz–40.0 GHz. With an elaborately designed broadband balanced matching circuit, the module is able to realize the power detection of the entire target frequencies with a dynamic range from –10 dBm to +10 dBm. The achievable detection accuracy is high to 0.1 dB. An external tunable resistance is provided for flexible detection within this dynamic range. Furthermore, the designed temperature compensation circuit enables measurement accuracy within ±1 dB over the full temperature range from –55 °C to +85 °C. Encapsulated by a 9 mm × 6 mm × 3 mm Kovar alloy shell, the module is both reliable and compact. It is also conducive to further integration.
2 Circuit design and simulation
2.1 Operating principle of the detection module
The basic principle of the MMW power detection circuit is as follows: The input signal is first rectified and then converted into a direct-current (DC) signal, which is generally implemented by diodes or other rectifiers. In order to realize stable DC power measurement, the rectified signal needs to be further filtered, where capacitors and inductors are used to eliminate high-frequency noise, thereby obtaining a smooth DC signal. A comparator is also included in the power detection circuit to compare the rectified DC signal with the predefined threshold.
Based on the principle of diode detector and broadband matching technology, the analog signal is converted into a digital signal. As shown in Fig. 1, the detection circuit mainly includes a detection unit (a detector diode with an input matching circuit) and a comparison processing unit (a comparator with a predefined threshold voltage). The MMW signal is first transformed to a low-frequency signal, and then compared with the predefined threshold via the comparator. Finally a detectable transistor-transistor logic (TTL) signal is output.
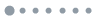
Figure 1.Schematic diagram of the detection circuit.
2.2 Diode detection circuit
As the most important component of the detection circuit, the detector diode plays a decisive role in its performance. The simplified diode equivalent model is shown in Fig. 2. It indicates that the corresponding overall performance is directly determined by the junction capacitance (Cj) and voltage sensitivity which is positively associated with the junction resistance (Rj). The junction capacitance (Cj) also has a significant influence on the operating frequency of the detection circuit. The larger the Cj value, the worse the corresponding high-frequency response [14]. This means that, to improve the detection sensitivity, the diode with low forward voltage drop and high speed is preferred during designing the high-frequency detection circuit, such as low barrier Schottky diodes. This diode equivalent model has been simulated in the Ka band by using the Advanced Design System (ADS) software. When the input power is 0, the simulated circuit diagram of the diode detection circuit is shown in Fig. 3. It can operate in the frequency range from 26.5 GHz to 40.0 GHz. Correspondingly, the simulated results of standing-wave ratio (SWR) and output voltage are shown in Fig. 4. It is obvious that the maximum of SWR is achieved at 33 GHz and it remains lower than 1.2 within the whole frequency band (26.5 GHz–40.0 GHz) owing to the balanced matching circuit customized for the detection circuit. This indicates that the characteristic impedance of the transmission line is well matched with those of the source and load, and the input signal is rarely reflected. Correspondingly, the output voltage is slowly increased from 575 mV at 26.5 GHz to 591 mV at 40.0 GHz, which satisfies the needs of this detection circuit.
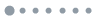
Figure 2.Simplified diode equivalent model.
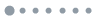
Figure 3.Simulated circuit diagram of the designed detection unit circuit for 26.5 GHz–40.0 GHz.
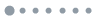
Figure 4.Simulated results: (a) SWR and (b) output voltage.
2.3 Temperature compensation circuit
Considering that the temperature fluctuation will affect the sensitivity of the diode and the reliability of the device, temperature compensation is necessary to eliminate its negative influence on the voltage and thus accurately detect the output voltage. Fig. 5 shows the change in the output voltage with the temperature at the frequency of 26.5 GHz when the input power is 0. The output voltage of the diode increases by about 20 mV from 630 mV to 650 mV when the temperature is increased from –55 °C to –30 °C, and then gradually decreases to 560 mV when further increasing the temperature to 85 °C. This result indicates that higher detection efficiency and output voltage can be obtained at a relatively lower temperature. It is also demonstrated that the temperature sensitivity of the diode has an obvious impact on the output accuracy of detection circuits in practical applications.
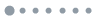
Figure 5.Output voltage curve as a function of temperature.
Thermistors are usually combined to realize temperature compensation when the output voltage is amplified. However, this kind of method has obvious disadvantages. The components inside the detector need to be screened during manufacturing, resulting in a large workload. In addition, the characteristics of the voltage buffer amplifier cannot better complementarily fit with that of the diode. The introduction of the buffer amplifier also makes it more complicated to miniaturize the circuit [16]. In this paper, the double-diode mutual compensation method is adopted, which is simple, easy to manufacture, and high-efficient for temperature compensation. Furthermore, this method also can effectively reduce the detection error of the output voltage caused by the temperature fluctuation. The schematic diagram of the proposed temperature compensation circuit is shown in Fig. 6. Considering the temperature fluctuation of the detector diode, a same diode was added to the out-of-phase end of the comparator. Thus these two diodes show a consistent change trend in the temperature. When the output voltage of the detector diode changes with the temperature, the threshold voltage at the out-of-phase end of the comparator also changes with a same trend. Therefore, the output voltage of the detection circuit remains relatively stable and is not affected by the temperature fluctuation.
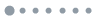
Figure 6.Schematic diagram of the designed temperature compensation circuit.
2.4 Cascading of unit circuits
The diode detection circuit, comparator, and temperature compensation circuit were cascaded and encapsulated by a Kovar alloy shell because of its good air impermeability. As a result, the volume of the proposed module is 9 mm × 6 mm × 3 mm. The surface of this shell was gilded to prevent corrosion, which makes it capable to be applied to harsh and complex environments. The micro-assembly process of hybrid IC was applied. The shell and the substrate were bonded with the gold-tin alloy solder. The substrate and components were bonded with conductive adhesive, and gold wire bonding was used for interconnection. This kind of hybrid IC constructed by discrete devices is applicable in high-reliability environments such as satellites. Such a small size is also desirable, which has been reduced by 1/4 compared with that of domestic hybrid IC devices [12].
Fig. 6 also shows that the MMW signal has been balanced and matched with the detector diode. The Schottky detector diode with small junction capacitance was selected. As low as 0.1 dB of power change can be detectable by such a detector and it has been verified by the engineering application, which will be shown in Section 3. The output voltage was compared with the threshold voltage VT2 at the out-of-phase end of the comparator. A high-speed comparator with a rising edge of 10 ns was selected. When the power was gradually increased to a certain value at a step of 0.1 dB, the output voltage was ~4 mV larger than the threshold voltage, and the output signal of the comparator was flipped from low-level TTL to high-level TTL, thus a warning was generated. The high-level output voltage has a driving ability, which can be adopted as the control voltage by directly connecting to the external switch or the enabling port of the digital integrated unit. The voltage at the out-of-phase end of the comparator in the circuit can be adjusted by an external tunable resistor RT. Correspondingly, the power in the dynamic range from −10 dBm to +10 dBm within the full frequency band can be successfully detected, which enables this detection module feasible to be widely used in engineering applications.
3 Implementation and test
The pictures of the designed module are shown in Fig. 7. When the voltage VT2 is 1.2 V, the results of the three-temperature test are shown in Fig. 8. It can be seen that the detection module achieves the detection accuracy of 0.1 dB in the 26.5 GHz–40.0 GHz band. It also indicates that the detection module has a measurement error of ±1 dB within the full temperature range benefitting from the proposed temperature compensation circuit.
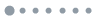
Figure 7.Pictures of the designed module: (a) internal layout diagram and (b) outline drawing.
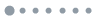
Figure 8.Results of the three-temperature test.
4 Conclusion and prospects
In this paper, a power detection module operating in a broad MMW band of 26.5 GHz–40.0 GHz was designed and realized with a low-barrier Schottky diode as a detector and a comparator used to determine the threshold. It can perform dynamic detection within the power range from –10 dBm to +10 dBm, which makes it applicable and feasible at different MMW frequencies. In the power module, a tunable external resistor was equipped. This not only enables the designed module can flexibly and dynamically detect the power, but also can be integrated at the system level, including synergetically cooperating with other sensors and communications modules, hence contributing to a more intelligent and efficient system. Moreover, the proposed module has the advantages of miniaturization and high reliability, and it is also easy to be integrated with other systems. It only has a small volume of 9 mm × 6 mm× 3 mm with less components and devices used. It was encapsulated by a Kovar alloy shell with good air impermeability, making it feasible to high-reliable environments such as satellites.
The miniaturization of such broadband power detection module is prospective and challenging. With the wide applications of 5G technology and the requirements of future communications systems for higher frequency bands, higher data rates, and smaller sizes, power detection modules are facing new demands and changes [19,20]. In future research, the following directions are worthy to be explored:
1) Intelligentization and self-adaption. With the rapid development of artificial intelligence and machine learning, the future power detection module will tend to be intelligent. The self-adaptive power detection algorithm can be automatically adjustable according to different working conditions, thus improving the detection accuracy and stability. Moreover, the machine learning technology renders more accurate power prediction and fault diagnosis and thus improves the system reliability.
2) Technologies related with high-frequency and MMW bands. With the increasingly increased communications frequency, the miniaturized broadband power detection module will be more and more attractive in the field of high-frequency and MMW bands. New high-frequency devices, antenna arrays, and signal processing technologies will bring more possibilities for the design of power detection modules.
3) New materials and new technologies. The application of new materials and technologies such as nanomaterials and quantum technology will provide a new way to improve the performance of power detection modules. For example, the high-sensitivity nanomaterials and high-precision quantum sensing are potential in the design of power detection modules to further enhance their performance.
4) Integration and multi-functions. The future power detection module is expected to be integrated with other MMW components more densely, contributing to a further reduction in the overall size and improvement in the system integration. Multi-functional design is another trend. The power detection module integrating with multi-functions, such as signal modulation and filtering, can effectively reduce the system complexity.
5) Standardization and applications. With the continuous development of technologies, standardization will become the key factor to promote the application of power detection modules. The development of unified test standards and performance indicators ensures that devices from different manufacturers can be compatible with different systems, driving the wide application of technology.
Disclosures
The authors declare no conflicts of interest.